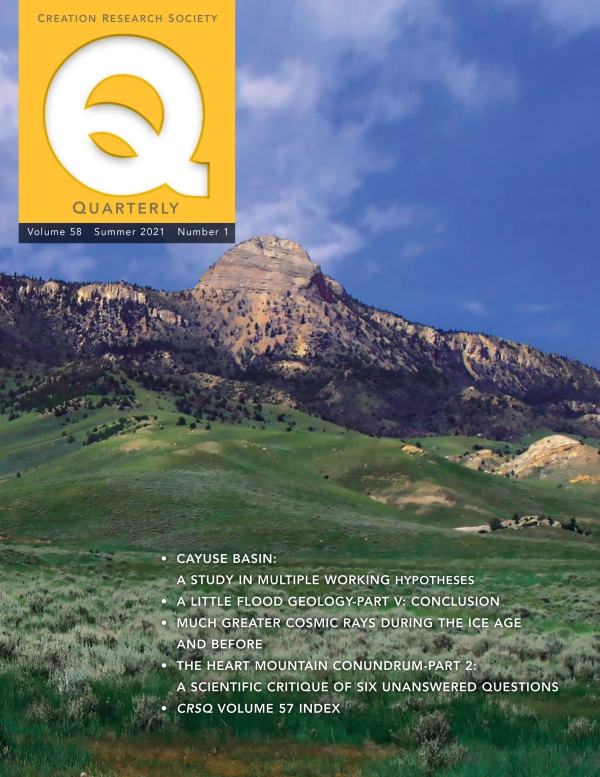
ABSTRACTS
The Heart Mountain Conundrum, Part 2: A Scientific Critique of Six Unanswered Uniformitarian Questions
John D. Matthews
Heart Mountain, Wyoming, USA, is a geological puzzle. “Paleozoic” carbonates overlie “much younger Mesozoic and Cenozoic” rock. The implication is that several mountain sized blocks broke from a detachment fault and slid up to 45 km. Part 1 of this series critiqued recent models that propose low-friction cushions to move the Heart Mountain blocks. This paper compliments that study by examining how the proposed movement started and was sustained over uneven terrain via seven questions raised by uniformitarian researchers. Current models, uniformitarian and creationist, cannot explain the location of Heart Mountain as a detachment and slide. Unless fresh ideas are forthcoming, the implication that these “Paleozoic” rocks of Heart Mountain are younger, not older, than the underlying “Mesozoic” rocks needs exploring
The Heart Mountain Conundrum, Part 2: A Scientific Critique of Six Unanswered Uniformitarian Questions
John D. Matthews
Heart Mountain, Wyoming, USA, is a geological puzzle. “Paleozoic” carbonates overlie “much younger Mesozoic and Cenozoic” rock. The implication is that several mountain sized blocks broke from a detachment fault and slid up to 45 km. Part 1 of this series critiqued recent models that propose low-friction cushions to move the Heart Mountain blocks. This paper compliments that study by examining how the proposed movement started and was sustained over uneven terrain via seven questions raised by uniformitarian researchers. Current models, uniformitarian and creationist, cannot explain the location of Heart Mountain as a detachment and slide. Unless fresh ideas are forthcoming, the implication that these “Paleozoic” rocks of Heart Mountain are younger, not older, than the underlying “Mesozoic” rocks needs exploring
Introduction
Heart Mountain, Wyoming, USA, is a large block of “Paleozoic” carbonates found atop “much younger Early Cenozoic (Eocene)” rock (Figure 1). Most researchers believe that this and several other mountain-sized blocks broke, with or without the involvement of volcanic rocks, and slid up to 45 km east-southeast (Pierce, 1957; Hauge, 1993). If not, it presents a significant local exception to the geological column. One path to a correct understanding of the Heart Mountain Detachment and Slide (HMD) is an analysis of proposed models of the event. In Part 1 (Matthews, 2021), one of the more intensely-studied aspects of the uniformitarian dilemma was examined—whether a low-friction cushion could explain the assumed sliding. Three models were examined: (1) selfgenerated CO2 , (2) high-pressure water, and (3) a carbonate melt. Without a low-friction cushion, friction during movement would have produced large amounts of breccia which is not there. The conclusion of that paper was none of those models met the physical requirements of breccia-free sliding.
This paper examines the issues and problems with other aspects of the supposed event; how movement was initiated, was sustained over uneven terrain, and was terminated. It centers on seven questions developed by uniformitarians. If models, uniformitarian or creationist, cannot answer these questions, then the visible order of the strata likely reflects their depositional order, providing a striking exception to the geological column. A more detailed study (Matthews, 2019) is available as a pdf from the author upon request.
Why Uniformitarians Think the HMD Occurred
The key reason geologists think Heart Mountain moved is stratigraphic—the relative dating of the overlying “Paleozoic” blocks and the underlying “Mesozoic” and “Cenozoic” rock. This requires a tectonic (or phreatomagmatic event), rather than a traditional sedimentary, explanation. Uniformitarians have suggested five additional reasons for the HMD event
1. Petrological evidence (Beutner and Gerbi, 2005), including certain layering interpreted as “microbreccia.” However, internal layering features show “sedimentary character that appear[s] to record deposition from suspension rather than friction.” (p. 724). This evidence is therefore equivocal. Layering within some of the blocks that moved show dips to the SE; normal extensional faulting (discussed below) would show an opposite orientation. Prostka (1978) suggested that this happened when the movements halted, with basal drag causing upper strata to tilt forward. But the breccia that should have been created is not present. The SE dip is in the down-slope direction and may be due to a distal pattern of sedimentation. This point about layering is therefore more consistent with a sedimentary hypothesis, not movement.
2. Beutner and Gerbi (2005) also note that groove casts, typical of those created by ice sheets, suggest sliding. These are significantly up-dip from White Mountain and Heart Mountain but not well documented down-dip. Furthermore, the dominant angle is only approximately that of the proposed direction of movement. Additionally, the range of angles of the grooves is about 40º (p. 731). This evidence is problematic, especially given the distance of movement
3. In the Beutner-Gerbi (2005) model, sliding occurred atop a low-friction CO2 cushion. The CO2 was generated from heating the carbonate at the base of the moving blocks to the point at which CO2 was released. This would have left lime, based on the endothermic reaction: Ca CO3 + heat >> CaO + CO2 Since large amounts of lime (CaO) are not present today, the model requires that, after the slide, lime recombined with local CO2 to return to its native (carbonate) state. Again, this point is equivocal, being an argument from an absence of evidence. Beutner and Gerbi (2005, p. 734) also note that there should be residual magnesium minerals (which do not re-combine with CO2 ) because the carbonate is not pure CaCO3 , but dolomite. Since these minerals are not present, no movement is a better explanation.
4. Igneous dikes are present in the blocks. These cannot be traced down into the rocks beneath them. This suggests that movement could have occurred, though it has not been possible to correlate the different sets (between the allochthons and the open surface) to determine the amount and direction of supposed movement.
5. There is no direct information available on relative radiometric dating which might clarify the question of movement. However, Clarey (2012) notes that the HMD post-dates the deposition of the Eocene Willwood Formation (which it lies upon) and pre-dates the volcanic-rich Wapiti Formation (which lies atop some of the HMD blocks). Using this relationship, and radiometric dates from the volcanic rocks, secular scientists have concluded that the movement on the HMD was early to middle Eocene (Clarey, 2012). But the longstanding vexed issue of leakage of the associated high-pressure cushion necessary for movement (Murrell, 1981; Matthews, 2016) of the South Fork system is not explained any more than there is an explanation for Heart Mountain’s movement (see Part 1). As such this interpretation is still equivocal.
The Uniformitarian Dilemma
Beutner and Gerbi (2005, p. 724) listed seven questions that puzzle uniformitarians regarding the supposed movement. These are refined versions of questions mentioned by Prostka (1978).
1. What caused a mass of rock of more than 1,100 km2 in area and several km in thickness to detach approximately along a bedding plane and slide on a slope of <2°, while spreading to cover more than three times its original area?
2. What initiated movement?
3. What caused the detachment to form near the base of the Ordovician Bighorn Dolomite rather than in weaker underlying rocks?
4. What was the rate of displacement?
5. What process or processes reduced friction to allow sliding on such a low slope?
6. What role did contemporaneous volcanism play?
7. Why is rock immediately below the slide surface so commonly undeformed?
The authors conclude:
…during the last century numerous geologists attempted to answer these questions. None of the resulting hypotheses [they list 11 ideas] attracted a quorum of supporters because evidence was not offered that unequivocally accorded with one hypothesis and negated others. (Beutner and Gerbi, 2005, p. 724)
If uniformitarians who advocate for the HMD discount prior models, and if subsequent uniformitarian models fail to explain the event (Matthews, 2021), then the logical next step is to ask if this phreatomagmatic event and sliding needs more scrutiny?
Question 5 was discussed in detail in Matthews (2021). Attention must now turn to the other six questions which largely relate to the tectonic issues. These should be related, in turn, to recent proposed models, of which Beutner and Gerbi (2005) is the most detailed available.
The Basic Model of Beutner and Gerbi
Beutner and Gerbi (2005) show, in their Figure 16, three snapshots in time illustrating their HMD dynamic model. Figure 2 encapsulates key aspects of nine distinct stages in HMD. I use “normal fault” nomenclature to explain the model, extending it to “overthrust” nomenclature when necessary
Stage 1
Detachment of what will move away from the “mother area” referred to directly in Beutner and Gerbi’s Question 1 (Q1)
Stage 2
The décollement—the freeing of the base of the part(s) that will slide (referred to indirectly in their Q1 but also part of their Q3 and Q7).
Stage 3
The fracture of the rocks in the “Toe” (monocline) area (referred to indirectly in Q1).
Stage 4
Injection of kinetic energy necessary to ensure movement (referred to indirectly in Q1, but also part of Q2). It may be related to Q6.
Stage 5
Low-friction sliding over the fault plane (part of Q4 and Q5)
Stage 6
Fragmentation of the moving mass into smaller portions (referred to directly, as “spreading,” in Q1). Or it could again be related to Q6. The timing of the fragmentation is not discussed but needs to be reconciled with their full model.
Stage 7
Ascent of the monocline; a point not mentioned in the questions but noted by Beutner and Gerbi (2005, p. 725) as a problem.
Stage 8
Moving over the “Mesozoic” layers (referred to indirectly in Q1).
Stage 9
Coming to a halt, being a point not mentioned in the questions but noted in Beutner and Gerbi (2005, p. 733) as needing an explanation.
Figure 2A shows a paleo-reconstruction of the area before sliding. The Crandall Complex is assumed to have been like a pillar rather than a dike. The HMD movement requires fractures to have developed at several locations so that portions of rock, which mainly consist of Palaeozoic carbonates (PC) overcapped by “Eocene” volcanic rocks (V-V), could have moved.
Stage 1 was the detachment (DT in Figure 2B). It required three distinct events. On the left of Figure 2B at the site marked “X,” faulting separated the back and side edges of the block from the mother mass. The footwall was left behind, while the right-hand side became the hanging wall. Stage 2 was the development of the nearly horizontal sliding surface, or the “décollement” (DC), literally the shearing within the dolomite itself 2.5 m above its base and the ungluing or unsticking of that same ‘Ordovician” carbonate from the lower layers in other locations. Aharanov and Anders (2006, p. 166) mention this strange position saying that it happened in a “non-descript bedding plane…. We observed virtually no deformation present in the rocks directly below the contact even at the scale of a single thin section.” For further comment on how it causes additional problems for the model, see the reference to the work of Hsu in Matthews (2019).
That allowed the block bordered by the hanging wall to slide to the right without disturbing the lower surface— the remaining thickness of dolomite and the “Cambrian” clastics (CC). The last event, Stage 3, formed the “Toe,” which is a fracture at the leading edge of the block (Figure 2B), at a low, but nontrivial angle abutting the “Mesozoic” clastics (MZ) at the monocline
To dismiss these essential stages as the product of some vaguely described “major volcanic or phreatomagmatic event” (Beutner and Gerbi, 2005, p. 734) is obfuscation. The closest model that could be offered to meet Beutner and Gerbi’s need is to imagine that highpressure water had been conveniently forced from depth at position “X,” at lithostatic pressure (around 80 MPa based on ~3 km of overburden). It would have had the potential to fracture the “Palaeozoic” carbonate and volcanics up to the surface thus initiating the “detachment.” Note that it had to be left of the Crandall Intrusive Complex (CIC) and so cannot be related to it. In fact, the preexistence of the proto-CIC suggests that it was already a geological valve relieving excessive underground pressures, which leads to the question of the need for a new “valve” so close to the CIC.
Though possibly sufficient to form the Stage 1 detachment, such water probably could not have simultaneously formed the “décollement.” That would have required sustained pressure much higher than lithostatic, so that the “hanging wall” was lifted and freed to move. Such water would have more likely moved vertically because, as Cosgrove (1997) reminds us, the minimum horizontal stresses are around 70% of the lithostatic pressure (i.e., ~56 MPa), though it could be as low as 40 MPa (Aharonov and Anders, 2006). For the décollement to have propagated horizontally from this point would have required an additional pressure of ~10 MPa at the tip, to part the formations, because of the natural cohesion of rock, ~80 MPa to overcome the lithostatic pressure, and an amount possibly up to 10 MPa to force the water from position “X” to the “Toe” along the gap of the décollement.
So, in total, the pressure at the fracture tip must have been around 100 MPa. But with a potential escape route for the fluid of just 3 km through a vertical fracture (requiring a pressure of no more than 56 MPa) rather than 30–40 km horizontally, there is no obvious explanation for the décollement to propagate to the “Toe.” Finally, Q3 invites a comment on why the décollement was not in the lower, weaker rock or, for that matter, why the assumed décollement was 2.5 m above the base of the dolomite (Q3). Also note that it would have had to cut across the pillar of the CIC and avoid riding up the strata at the Blacktail Thrust (Figure 2A).
Even if these unlikely events occurred, the remaining stages are equally problematic. First, the HMD must have happened quickly; otherwise, the fractures would have healed due to natural earth stresses, migration of magmatic fluids, and their potential to re-cement fractures. Hot, high-pressure magmatic water might have created fractures, but it could also heal them, being rich in dissolved minerals that would precipitate as pressure and temperature fell. Sliding requires a mechanism that explains a long-term low-friction zone, and the initial propulsion of the blocks. Also, kinetic energy has to be injected into the system for most models (Stage 4). Otherwise, we cannot move past the stage illustrated in Figure 2C
Furthermore, how and when the initial block divided into smaller blocks (Stage 5) is not addressed in any model. Some of these sub-blocks only “travel” over a thin dolomite layer, such as White Mountain, but Heart Mountain proper rode up the monocline, then slid atop the “Cenozoic” rock (Figure 2D).
Figure 2C shows where the portions of the hanging wall move right, over regions of very low friction on the décollement (Stage 6). Some models claim that the force of gravity down the <2º slope is sufficient for movement, but this is less than 3.4% g (0.3m/s2 ). Furthermore, there are the forces needed to have overcome the stratigraphic and physical rise (Stage 7) at the “Toe,” near the monocline. The physical rise would have been 300–500 m, based on the mapping in Prostka (1978) and a basic consideration of how the monocline and subsequent unconformity would have formed. This “wastes” about half the value of the already low slope as a mechanism for movement. Furthermore, we cannot explain why these terrain irregularities did not break up Heart Mountain. In fact, the fragmentation shown in Figure 2C is more likely to have occurred after the monocline ramp than before it
Q4 is effectively linked to Q5, and the low-friction zone. Models of it were critiqued in Matthews (2021). All were deficient. Stage 8 did not involve White Mountain, since it did not climb the monocline.
Figure 2D shows where the sliding blocks came to a halt (Stage 9), with Heart Mountain resting on “Cenozoic” rocks (CZ). As this happened, the hypothetical low-friction cushion would have disappeared. Rock-to-rock contact would have slowed and stopped motion. This brings us back to Q7, and the lack of deformation in the rocks
Beutner and Gerbi (2005) briefly mention how a fast-moving block (40 m/s given their assumptions or Mach 4 from Matthews, 2021) could have stopped without deformation, given the absence of a brecciated zone and an undeformed underlying layer (their Figure 3). To resolve the dilemma, they write that there was an “abrupt cessation [of] movement due to lateral contact between blocks” (p. 733) based on an analogy with the Elm, Switzerland (1881) rockslide, which an eyewitness claimed ceased dramatically. But their analogy is totally inadequate. Elm was a mining accident. The mass of rock in it was minuscule compared to HMD-S, and the velocity, significantly smaller. There were several pulses of movement. The Elm Slide was on a mountain side substantially steeper than HMD. Finally, the way energy is lost in a granular mass of moving rock (Elm) is a very different process for a solid mass (Kilburn, 2001). Their confusion shows in their final words:
As the slide slowed and thinned, however, it would lose its cushion of gas and at some point frictional drag would dominate, and motion would abruptly cease. (Beutner and Gerbi, 2005, p. 735, emphasis mine)
It surely is an ad hoc suggestion with a touch of obfuscation (by trying to link it back to Elm) that such a large mass can come to a halt abruptly without brecciation and significant deformation, regardless of the velocity. And what “thinned”? Based on normal rock-to-rock friction, the mountain would have slid about 150 m with thick beds of breccia forming as the low-friction cushion disappeared. If it had been moving at Mach 4 (given more realistic assumptions), it would have hardly survived the monocline, and if it did, would have slid out of the basin area when the cushion ceased and almost certainly shattered in the process.
The Model of Aharanov and Anders
Other models share many of the same faults. Aharonov and Anders (2006), in a short paper, do not mention the seven questions, but implicitly attempt to answer parts of Questions 1, 2, and 5. They suggest that dikes, emerging from depth at high pressure, on what would become the fault plane, could explain how the slide started on a near-zero-friction surface of water. Their calculations of rock mechanics, temperature changes and overpressure show that, with the right series of assumptions, the pressure of the interstitial water could have been raised by the Skempton effect to the lithostatic value.
The Skempton effect (Jaeger et al., 2007) is the way pressure on rock is partially transferred to interstitial water.If they were correct, the block could then start to move down the small slope without the need for adding kinetic energy, as Beutner and Gerbi require. But with a slope of <2º the mass would have moved slowly. The high-pressure water would leak out of the edges, so the key problem to be answered is how the slide continued, travelled up the monocline and across the “Mesozoic” rock. The block would have moved away from the high-pressure stress point of the dikes and onto an unpressurised surface. Any high-pressure water that emerged onto the open surface would have disappeared while the block accelerated, resulting in rock-to-rock contact, and the inevitable halt
There is a further problem with the model. The very mechanisms that increase the pressure in the interstitial water would have hampered the essential detachment (Stage 1) by raising the horizontal stress in the rock. So, in total, the model does not do justice to the essential requirements for a detachment or a 45-km slide.
The Model of Craddock et al.
Craddock et al. (2009) wrote about White Mountain (about 30 km “upstream” of Heart Mountain). Since White Mountain was initially part of the HMD event, its study was done to cast light on HMD, and indirectly provide support for interpreting HMD as a slide.
This model simply assumes detachment (Stages 1–3). It posits the block moving at an unknown velocity that can be calculated by assuming that the low-friction cushion on which it moved was a layer of molten carbonate. The heat to melt it came from the friction of sliding, which begs the question of how the motion began. So, Questions 1 to 3 are ignored, Q4 is answered by assuming that the slide took place (circular reasoning). Q5 is answered by a less-thansophisticated heat transfer calculation which ignores the inevitable heat lost to the base plate, and Q6 and Q7 are ignored. When the corrected equation is used, the block is predicted to have a negative velocity. See Matthews (2019).
While the paper of Aharanov and Anders (2006) appeared shortly after that of Beutner and Gerbi (2005) (though they make a brief reference to it), the paper of Craddock et al. (2009) appeared sufficiently later for the authors to re-assess the models of Beutner and Gerbi, and Aharanov and Anders. But there was no such assessment. The contrast in the models is so stark that the only natural conclusion is that both previous models were thought unworkable by Craddock et al. (2009). Their model fares no better.
Searching for Analogues
In a later paper, Craddock et al. (2012) compared the apparent movement of White Mountain to the Mt. St. Helens eruption. However, the block sliding was much more intense; they do not have a good analogue for HMD because (p. 436, emphasis mine):
The relationship of hanging wall displacement to fault gouge generation, and the presence of vertical, 120 m, fault gouge injectites, makes the Heart Mountain slide anomalous to all fault systems, especially as this fault system had only one episode of motion
Furthermore, this paper did not shed more light on mechanisms, where we might have expected them to try and integrate earlier ideas of Beutner and Gerbi (2005), Aharanov and Anders (2006), and Craddock et al. (2009). White Mountain is on a base of “Ordovician” carbonate ultracataclasite (>90% matrix), different from the “Cenozoic” base of Heart Mountain. So, there is no new information on how HMD might have occurred. Their quote (above) can be read as implying that HMD was not a slide.
In conclusion, all uniformitarian models are inadequate. In fact, those authors present evidence (and not just through their list of unanswered questions) that the detachment and slide did not take place. Is it possible that the distinct Flood paradigm can generate a creationist model that solves those problems?
Creationist Responses to HMD as a Slide
At least two creationists (Garner, 2011; Clarey, 2013) identify secular studies that conclude that Heart Mountain moved at high speed. They note that this admission of a major catastrophe by uniformitarians is surprising in itself but do not challenge the details of the studies.
Oard (2010) dug deeper and reviewed three of Beutner and Gerbi’s (2005) seven questions. He accepts that a detachment and slide occurred, but subaqueously, during the Flood. He uses that environment to help explain the necessary catastrophic release of energy to get HMD detached and moving (thus addressing, but not quantifying, Q1 and Q2). A submarine slide would address Q5, the water providing a basal cushion for the slide. This short paper was, of course, written before several of the secular papers analyzed above and is not detailed.
Developing Oard’s (2010) model further is hampered by the following. First, a block moving under water, particularly when propelled by gravity, has less force on it (because buoyancy acts in the opposite direction to gravity). So, the ratio of frictional drag to gravitational forces is unchanged. Motion cannot be enhanced by a submarine slide. Second, there is an additional viscous drag due to the water surrounding the moving block. Thus, a rapid underwater slide is not realistic. Q3 (the detachment mechanism) is not answered by Oard (2010) and Q4 is answered by the timescale of the Flood (but not quantified by physics). In a model with a more-than-adequate supply of lubricant (water), the velocity does not have to be fast. However, there is no physical model that will explain the lubrication that needs to be sustained at low or high speed without postulating a supply of high-pressure water emerging from depth moving exactly beneath HMD on its journey to its final resting place. With viscous drag capable of bringing it to a halt rapidly when the lubrication ceases, there would probably be less brecciation than predicted by any of the uniformitarian models described above. Q7 remains unanswered because a block moving underwater still has the potential to deform the underlying strata. So, although there are some new ideas here, a robust Flood-explanation for a slide has yet to be worked out.
A Brief Summary
Geologists have been baffled by Heart Mountain for over 100 years (Hauge, 1993). Based on their relative age dating, it appears to have detached and slid up to 45 km in the early to middle “Eocene” (Clarey, 2012). Beutner and Gerbi (2005), prompted by Prostka (1978) posed seven questions that need answering before HMD can be categorically interpreted as a slide. There have been several attempts to explain HMD by analogies to smaller slides. However, the circumstances were sufficiently different as to be of no help.
Beutner and Gerbi (2005) proposed a basic model that focused on just one of their questions—the low-friction cushion. Their proposal, using CO2 generated from the dolomite, was shown to be deficient in Matthews (2021). Their failure to address the other six questions leaves their model unsustainable. Aharanov and Anders (2006) attempted to answer two of the questions. Although the paper appeared after Beutner and Gerbi (2005), it does not build on their model, thus it indirectly challenges it. However, they offer no mechanism for sustaining a low-friction zone to keep the rock mass moving away from the initial high-pressure water source. They fail to address four of the questions, leaving their model seriously deficient. The Craddock et al. (2009) model requires a calcite melt-layer for a cushion. But it must be present before movement starts. So, it suffers from similar problems as the Aharanov and Anders (2006) model. It does not offer answers to six of the questions. Likewise, Oard’s (2010) creationist model of submarine sliding fails to answer most of the questions, and thus fails the test.
In summary, the failure of any model to adequately answer even one of the seven questions leads to the possibility that HMD was not a detachment and a slide. The nine stages of the proposed process are untenable. If true, it presents a stunning local exception to the geological column. Other papers (e.g., Reed and Oard, 2006; Matthews, 2011) show many other geographical exceptions to the column. Thus, creationists must seriously consider that the geological column may not be valid for modeling the Flood and indeed should offer this evidence as a contra-indicator of evolution as noted originally by Whitcomb and Morris (1961).