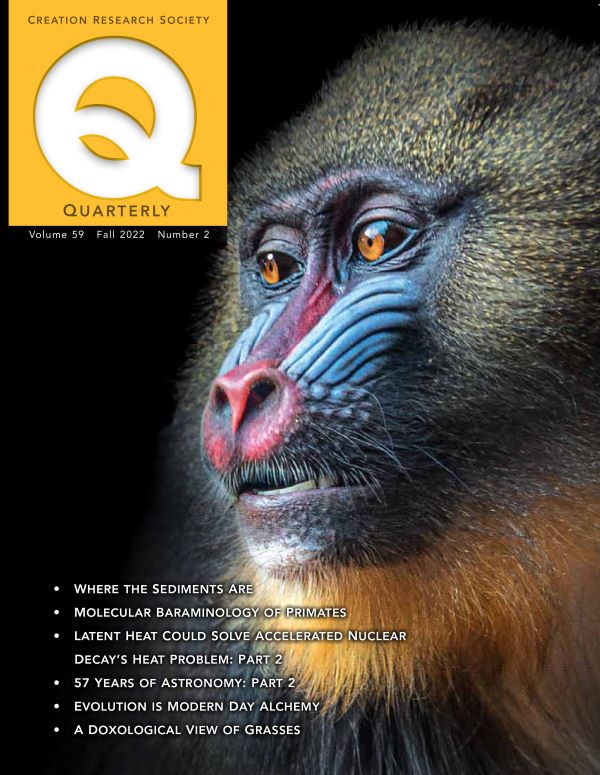
ABSTRACTS
Molecular Baraminology of Primates
Matthew Cserhati
Twenty-six species of primates were examined by the Gene Content method, and 93 mitochondrial genome sequences were downloaded from the NCBI database, aligned, and analyzed. In total, 96 species were studied, about half of all primates. The Gene Content method predicts at least six putative primate holobaramins: cercopithecines, colobines, New World monkeys, lemurs, great apes, and humans. The mitochondrial DNA analysis predicts sixteen groups, which are likely monobaraminic lineages within the groups predicted by the Gene Content method. This study predicted a larger number of groups compared to a previous study of primates and other vertebrates. Interestingly, examination of the newly sequenced mitochondrial genome of Homo heidelbergensis places it within the human holobaramin, in agreement with previous studies that included it based on morphological data alone. The human holobaramin also clearly separates from all other primate species, even its alleged closest relatives, the great apes. Hylobatidae is possibly a seventh primate group, and Pongo (orangutan) is a possible eighth group.
Molecular Baraminology of Primates
Matthew Cserhati
Introduction
There are 194 species of living primates (Stringer and Andrews, 2011), which range in size from the tiny, palm-sized Moholi bush baby (Galago moholi) (around 6 oz. in weight) to the large gorilla (Gorilla gorilla) (up to 227 kg in males) and are (excluding humans) found on all continents except Australia and Antarctica. Primates are classified into two suborders (see Figure 1), 1. Strepsirrhini, such as lemurs, lorises, and galagos, and 2. Haplorhini: tarsiers and anthropoids. Anthropoids can be classified as either flat-nosed monkeys (Platyrrhini), otherwise known as New World monkeys, and Catarrhini, or Old World monkeys with downward-looking nostrils. Platyrrhini is subdivided into five families: Callitrichidae (marmosets and tamarins), Cebidae (capuchins and squirrel monkeys), Aotidae (owl monkeys), Pitheciidae (titis, sakis, and uakaris), and Atelidae (howler monkeys and spider monkeys). Catarrhini is subdivided into two superfamilies, Cercopithecoidea, which has one family, Cercopithecidae (including langurs, macaques and baboons), and Hominoids. Hominoids are further classified into two families Hylobatidae (gibbons), and Hominidae. Hominidae is subdivided into the subfamilies Ponginae (orangutans), Gorillinae (gorillas), and Homininae. Hominines are made up of the tribes Panini (chimpanzees, genus Pan) and Hominini, otherwise known as hominins, made up of the genus Homo (humans) (Park, 2009; Stringer and Andrews, 2011; Gebo, 2014).
The human species (Homo sapiens) is unique among all extant primates, even all extant animals, in that we alone were created in God's image (Genesis 1:26-27) (Lightner and Cserhati, 2019). Archaic humans, such as Neanderthals (Homo sapiens neanderthalensis), Denisovans (Homo sapiens denisova), and other possible groups are also members of humankind (Lubenow, 2004; Savanne, 2014; Rupe and Sanford, 2017; O'Micks, 2018a, 2018b). Based on their morphological characteristics, several other fossil species have also been considered to be members of the human holobaramin. These include Homo erectus, Homo heidelbergensis, Homo naledi, and Homo rudolfensis (Wood, 2010; Line, 2013; O'Micks, 2016; Wood, 2016; Rupe and Sanford, 2017. Some of these placements are controversial, so molecular data provide another line of evidence that can be useful in classifying these taxa. With the mitochondrial genome sequenced for Homo heidelbergensis (Meyer et al., 2014) we now have the opportunity of placing this fossil taxon into its proper category with even more confidence.
Almost all primates are characterized by grasping feet with a divergent hallux, presence of nails on most digits, an elongated calcaneus, hind limb locomotor dominance, grasping hands with opposable thumbs, forward rotation of the closely set eyes, large brain size, relatively long gestation compared to body weight, slow fetal growth, prolonged life history, and a loss of an incisor and premolar from the tooth row (Stringer and Andrews, 2011).
Until now, the creationist literature has dealt mainly with the analysis of groups or individual hominins or australopithecines. For example, the families Cercopithecidae (Hartwig-Scherer, 1993), Galagonidae (Wood, 2008), and the subfamilies Gorillinae (Hartwig-Scherer, 1998) and Ponginae (Hartwig-Scherer, 1998) have also been studied individually. A more comprehensive study of the order Primates has been lacking. With proteomic data available from the NCBI database, a comprehensive molecular baraminology study can be done using the Gene Content (GC) method of O'Micks (2017).
Materials and Methods
Gene Content Method Analysis
The whole proteomes of 26 primate species were downloaded from the NCBI database. Monodelphis domestica and Mus musculus were used as outlier species. Protein predictions were made for Neanderthal and Denisova using the Augustus gene prediction software. Proteins were assigned to OrthoMCL clusters following the GCM protocol (Stanke and Morgenstern, 2005; Fischer et al., 2011; O'Micks, 2017). The number of proteins and the number of proteins mapped to orthologs for each species were noted and can be seen in the 'species' tab of Supplementary File #1, which also contains the results for this analysis. It is available on Zenodo at https://zenodo.org/record/6635435#.YqWDGOzMLrc.
Mitochondrial DNA Analysis
The mitochondrial genome sequences of 92 primate species were downloaded from the NCBI Organelle database at ncbi.nlm.nih.gov/genome/browse#!/organelles. Monodelphis domestica was used as an outlier. One sequence each for Gorilla gorilla gorilla and for Pan paniscus was excluded because it was a duplicate. The alignment of these mtDNA sequences was done using the CLUSTALW2 alignment tool (Thompson et al., 2002). The results of the mtDNA analysis are available in Supplementary File #2.
Results and Discussion
Results of the GC Method
The JCV matrix (see Supplementary File #1) has a Hopkins measurement value of 0.793, which is good quality for clustering. An elbow plot (Supplementary Figure 1) generated from the matrix shows an optimal number of clusters at k=12. The GC method was run to search for twelve baramins according to protocol (O'Micks, 2017). The species classification and the statistics for the predicted baramins can be found under the tabs 'species classification' and 'stats' in Supplementary File #1. It is available on Zenodo [https://zenodo.org/record/6635435#.YqWDGOzMLrc].
On the heat map in Figure 2, several clusters of species can be seen. Group 1 (red) includes four species of New World Monkeys: Aotus nancymaae, Callithrix jacchus, Cebus capucinus imitator, and Saimiri boliviensis boliviensis. The last three belong to the family Cebidae (marmosets, tamarins, capuchin monkeys, and squirrel monkeys). Aotus nancymaae (Ma's Night Monkey) belongs to the family Aotidae (owl monkeys). However, according to newer primate taxonomies based on the phylogeny of the epsilon globin and interphotoreceptor binding protein (IRBP) intron 1 (Schneider and Sampaio, 2015) the genus Aotus is now included in the family Cebidae as well, meaning that all species used in this study can be considered to represent a single family. This group has a p-value of 1.4E-10, and a mean JCV of 0.947. Hybrids have been reported between species from the genus Callithrix and Callicebus, which belong to the families Cebidae and Pitheciidae (Neusser et al., 2005; Fantini et al., 2011).
Group 2 (yellow) makes up the largest group, in the middle, with eight species of cercopithecine Old World monkeys, with a p-value of 3.84E-28, and a mean JCV of 0.96. These include species from the genera Cercocebus, Chlorocebus, Macaca, Papio, and Rhinopithecus.
Group 3 (olive green) is made up of another group of Old World monkeys, the colobines, with two species, Colobus angolensis palliates (Angolan black-and-white colobus monkey), and Mandrillus leucophaeus (the mandrill). Interestingly, there have been two recorded hybridizations between members of the genus Mandrillus and Cercocebus from the previous group (Van Gelder, 1977).
Humans (group 4, light green) form a very compact cluster, visibly discontinuous with all other primate species, with a p-value of 1.8E-07, and a mean JCV of 0.979. This is in accordance with results from a previous molecular baraminology study performed on mammals by Lightner and Cserhati (2019). The present study compared 26 primates with one another, as opposed to 21 in the previous analysis. Both studies underscore the uniqueness of humans compared to all other animals, even other primates, which are those animals to which humans are most similar. Whereas humans have a JCV of 0.979±0.005, this drops down to 0.8±0.001 when the JCV are compared between humans and the great apes. This means that if we add the great apes to the human baramin, we get meaningless results since the mean JCV is greatly reduced. If we calculate a z-score on the two JCV distributions, we get a value of 16.01, which means that the two distributions are very highly dissimilar.
Group 5 (bluish green) is made up of the great ape species: the gorilla (Gorilla gorilla), and two chimpanzee species (Pan paniscus and Pan troglodytes). These three species show discontinuity with all other species, with a p-value of 2.4E-12 and a mean JCV of 0.956.
Two species of lemurs, the Gray Mouse Lemur (Microcebus murinus) and Coquerel's sifaka (Propithecus coquereli) make up cluster 6 (cyan), with a mean JCV of 0.951.
Several individual species could not be assigned to any cluster. These include Carlito syrichta (Philippine tarsier, orange) and Otolemur garnettii (Small-Eared Galago, purple), Nomascus leucogenys, the White-Cheeked Gibbon (dark purple), from the family Hylobatidae, as well as Pongo abelii (the Sumatran orangutan, magenta). Monodelphis domestica (blue) and Mus musculus (dark blue), the two outlier species also cluster separately from all of the other species.
Mitochondrial DNA Analysis
Putative clusters were predicted based on aligning the mtDNA sequences of 92 primate species and an outlier, Monodelphis domestica. The silhouette plot in Supplementary Figure 2 shows an optimum value at k=16. Thirteen of the sixteen clusters were comprised of at least 3 members and were statistically significant with a p-value less than 5%. The mtDNA sequence similarity cluster had a Hopkins clustering value of 0.897. The list of species used in this study, the mtDNA identity matrix, the putative clusters, and the statistics for the predicted baramins can be found in see Supplementary File #2.
The sixteen clusters are as follows: the first cluster (in red in Figure 2) consists of five species from the genus Rhinopithecus (snub-nosed monkeys): three Rhinopithecus bieti individuals, Rh. strykeri, Rh. roxellana, Rh. brelichi, and Rh. avunculus. This is a genus of Old World colobine monkeys.
The second and third clusters (orange and dark orange) are made up of three species from the genus Pygathrix (douc langurs): Pygathrix cenerea, Pygathrix nigripes, and Pygathrix nemaeus. This is a genus of Old World colobine monkeys.
Nine species make up cluster 4 (yellow): Simias concolor (the pig-tailed langur), Nasalis larvatus (the proboscis monkey), Presbytis melalophos (black-crested Sumatran langur), Trachypithecus johnii, Trachypithecus vetulus, Procolobus verus (olive colobus monkey, or Van Beneden's colobus), Semnopithecus entellus (the northern plains gray langur), Piliocolobus badius (western red colobus), and Colobus guereza (mantled guereza). Some researchers prefer to put the first two species into the same genus (Groves, 1970). Whittaker et al. (2006) also came to the same conclusion based on similarities between cytochrome b and several RNA genes. These nine species in cluster 4 are all colobine monkeys. An analysis of 54 genes covering 35 Kb of nuclear DNA (Perelman et al., 2011) places T. vetulus within the genus Semnopithecus, thus explaining the incongruency of why this species is seemingly misplaced from the next group, Trachypithecus. This might be true of T. johnii, another misplaced Trachypithecus species. Indeed, based on an analysis of a 573 bp segment of the mitochondrial cytochrome b gene, T. johnii forms a clade with the South Indian form of Semnopithecus entellus (Osterholz et al., 2008).
Group 5 (yellow-green) consists of eight species of Old World colobine monkeys, namely lutungs (leaf monkeys) within the genus Trachypithecus (T. cristatus, T. francoisi, T. germaini, T. hatinhensis, T. obscurus, T. pileatus, T. poliocephalus, and T. shortridgei).
Group 6 (light green) consists of six Chlorocebus species: Chlorocebus aethiops, Ch. cynosuros, Ch. djamdjamensis, Ch. pygerythrus, Ch. tantalus, and Ch. aethiops x Ch. pygerythrus. Here the letter 'x' between two species names denotes a new hybrid species which is a result of the cross between the two species that make up the hybrid's name. These are all cercopithecine monkeys. A seventh group consists of only Ch. sabaeus. The reason that this one species of Chlorocebus separates from all the rest may be due to mtDNA heteroplasmy, which is known to occur in this genus (Rensch et al., 2016).
Group 8 (light cyan) includes: Cercopithecus diana, C. albogularis, C. mitis, C. lhoesti, Erythrocebus patas, and Allenopithecus nigroviridis. These are all species of cercopithecine monkeys.
The ninth and tenth cluster of primate species (cyan and blue) includes seventeen species of macaques (genus Macaca). Macaques are cercopithecine monkeys and have a widespread geographic distribution, and live in places such as deserts to rainforests, from sea level to mountains, from North Africa to Southeast Asia. This genus might be split up into two groups because they might represent two monobaramins. Members of the first group are: Macaca arctoides, M. nigra, M. radiata, M. silenus, M. sinica, M. sylvanus, and M. tonkeana, whereas members of the second group are: Macaca assamensis, M. cyclopis, M. fascicularis, M. fuscata, M. leonina, M. leucogenys, M. mulatta, M. mulatta vestita, M. nemestrina, and M. thibetana.
Previous studies have usually discovered four groups of macaques (the fascicularis, silenus, sinica, and sylvanus groups). The membership of these groups varies as to which region of the mtDNA is under study, be it NADH4 and several tRNAs, the 12S and 16S ribosomal regions, the 3.1 kb TSPY and the SRY regions, the 2322 bp region of 12S, tRNAGlu, COI, COII and COIII, or the Alu elements of 358 loci, or the region around the COII gene (Rahim et al., 2014). However, since these studies did not examine the entire mtDNA, they remain inconclusive.
Group 11 (darker blue) is made up of five species from two cercopithecid genera: Cercocebus (mangabeys) and Mandrillus (forest baboons or mandrills). These are: Cercocebus atys, C. chrysogaster, C. torquatus, Mandrillus leucophaeus, and M. sphinx. Liedigk et al. (2014) created a multiple alignment of the mitochondrial genomes of papionins (Macaca, Mandrillus, Cercocebus, Lophocebus, Theropithecus, Rungwecebus, and Papio). They found that Cercocebus + Mandrillus forms a clade amongst papionins. The present results reinforce previous molecular results which show that drills are more closely related to mangabeys from the genus Cercocebus as opposed to a previously held view grouping them together with baboons (Disotell, 1994; Fleagle, 1999). In fact, Fleagle and McGraw (1989) suggest that similar jaw, fore- and hindlimb morphology between Mandrillus and Cercocebus allow for a foraging lifestyle on the forest floor. The previously mentioned study be Perelman et al. (2011) also found the Cercocebus-Mandrillus clade to be monophyletic (Devreese and Gilbert, 2015).
Six species from the genus Papio make up group 12 (darkest blue), as well as two species from two other genera. These are Papio anubis, P. cynocephalus, P. hamadryas, P. kindae, P. papio, and P. ursinus. These monkeys are also cercopithecids. The two other species are Lophocebus aterrimus (black-crested mangabey) and Theropithecus gelada (gelada). These papionid monkeys form a clade together based on the molecular analysis of 57 loci (Pugh and Gilbert, 2018). According to these same authors, the genera Cercocebus and Mandrillus form another clade. This clade was also found by Liedigk et al. (2014) in a multiple mitochondrial genome alignment. A phylogenetic tree based on the testis-specific Y-encoded protein (TSPY) shows that M. leucophaeus and M. sphinx intermingle with three species of Cercocebus, strongly suggesting that the species in these two genera belong to the same holobaramin (Kim and Takenaka, 1996; Harris, 2000).
Group 13 (dark purple) consists of three gorillas (Gorilla gorilla gorilla, G. gorilla and G. beringei) and two chimpanzee species: Pan paniscus and P. troglodytes.
The next group (14, purple) is made up of four individuals belonging to the genus Homo: Homo heidelbergensis, H. sapiens, H. sapiens neanderthalensis, and H. sp. Altai (Denisovan). This is a very interesting result, in that there is now molecular evidence to support the human status of H. heidelbergensis, which until now was classified as human based only on morphological traits (Line, 2013). It also reinforces the results that Neanderthals and Denisovans are both human.
Two species of orangutan make up group 15 (light purple), Pongo abelii and P. pygmaeus. These two species have been known to hybridize (P. abelii x pygmaeus) (Botting and Bastian, 2019).
Group 16 (magenta) is made up of eleven species from four genera: Hoolock (hoolock gibbons), Hylobates (gibbons), Nomascus (gibbons), and Symphalangus (siamangs): Hoolock hoolock, H. leuconedys, H. leuconedys x H. tianxing, H. tianxing, Hylobates agilis, H. lar, H. pileatus Symphalangus syndactylus, Nomascus gabriellae, N. leucogenys, and N. siki. All these species belong to the family Hylobatidae.
The outlier, Monodelphis domestica (white) shows discontinuity with all other species.
Summary and Conclusion
Table 1 summarizes the overlap in the results between the Gene Content method and the mtDNA analysis. As we can see, the Gene Content method found four groups (colobines, cercopithecines, great apes, human) also found by the mtDNA analysis. Each of these four groups, however, match several groups found by the mtDNA analysis. One group found by the mtDNA analysis (Hylobatidae) was not found by the Gene Content method. New World monkeys and lemurs were found by the Gene Content method but not by the mtDNA analysis.
What do these results mean? They most likely indicate that primate molecular baraminology has discovered seven simiform primate kinds: colobines, cercopithecines, great apes, New World monkeys (Cebidae), great apes, gibbons, and humans. The previous study by Lightner and Cserhati (2019) predicted only four primate groups: humans, great apes, Old World monkeys, and New World monkeys. This previous study only examined 21 species, among other vertebrate groups, whereas the present study examined 98 species.
The fact that the mtDNA analysis uncovered a larger number of groups could be because these groups are monobaraminic lineages within the corresponding larger group found by the Gene Content method. Indeed, the genera Pygathrix, Macaca, and Chlorocebus were each split into two groups. Further, two species of Trachypithecus cluster with Semnopithecus, in separate group from other Trachypithecus. Mitochondrial DNA analysis can only go so far in providing congruent results. Factors, such as heteroplasmy or higher mutation rates may obscure baraminic relationships based on mtDNA analysis.
The Gene Content method provides a more holistic view of baraminic relationships as opposed to the mtDNA study which examined only a small fraction of the genome. Interestingly, both the Gene Content method and the mtDNA analysis placed gorillas and chimpanzees into the same baramin. This may seem unusual due to the size disparity between these two species, however, such large size differences exist in other kinds, such as the cat kind (lion and house cat). Humans clearly separate from all other primates in this study, including their alleged closest relatives, the great apes, based on both the GC method as well as mitochondrial DNA analysis. This highlights their unique position within creation. Lastly, a very interesting result shows that based on molecular data, Homo heidelbergensis is likely a member of the human holobaramin (Cserhati, 2022), confirming what many creationists had suspected, based on morphological analysis. With the isolation of genetic material from even more fossil hominins our picture of baraminic relationships within primates is becoming more complete.
Acknowledgments
This paper was written as a part of CRS grant #62.
Tables
Table 1. Comparison of results between the Gene Content method and the mtDNA analysis. Names in parentheses are present in one analysis but are missing from the other.
Gene Content method
|
mtDNA analysis
|
Colobines |
Pygathrix (2 groups) |
Rhinopithecus |
|
Simias+Nasalis+ Trachypithecus+Semnopithecus+ Colobus+Piliocolobus+Presbytis+Procolobus |
|
Trachypithecus |
|
Colobus+Mandrillus
|
Cercocebus+Mandrillus |
Cercopithecines
|
Macaca (2 groups) |
Papio+ Lophocebus+Theropithecus |
|
Chlorocebus (2 groups) |
|
Allenopithecus+Cercopithecus+Erythrocebus |
|
Great apes |
Gorilla+Pan |
Orangutan |
Pongo |
Human |
Homo sapiens, Neanderthal, Denisovan, Homo heidelbergensis |
(Gibbons) |
Hoolock+Hylobates+Symphalangus+ Nomascus |
New World monkeys, family Cebidae: Aotus, Callithrix, Cebus, Saimiri |
(New World Monkeys) |
Figures
Figure 1. Taxonomic tree of primates. Primates are divided into Strepsirrhini and Haplorhini, which are classified as Tarsiiformes (tarsiers), Platyrrhini (New World monkeys) and Catarrhini (Old World monkeys). Catarrhini is made up of cercopithecoids and hominoids, which themselves are made up of Hylobatidae (gibbons) and Hominids. This latter group is made up of gorillas and orangutans and hominins (chimpanzees and humans). The human species (Homo sapiens) is unique among all primates.
Figure 2. Heatmap showing putative baraminic relationships between primates based on analysis by the Gene Content method. Darker, redder colors correspond to higher Jaccard Coefficient values, corresponding to species showing significant continuity with one another. Lighter, yellower colors correspond to lower Jaccard coefficient values denoting species from two separate baramins.
Figure 3. Heatmap showing putative baraminic relationships between primates based on analysis of the mitochondrial genome. Darker, redder colors correspond to higher sequence similarity values, corresponding to species within the same baramin. Lighter, yellower colors correspond to lower sequence similarity values suggesting species from two separate baramins.
References
Botting, J., and M. Bastian. 2019. Orangutans (Pongo pygmaeus and Hybrid) and gorillas (Gorilla gorilla gorilla) modify their visual, but not auditory, communicative behaviors, depending on the attentional state of a human experimenter. International Journal of Primatology 40(6):DOI: .
Cserhati, M. 2022. Baraminic placement of Homo heidelbergensis based on molecular data. Creation Research Society Quarterly, accepted.
Devreese, L., and C.C. Gilbert. 2015. Phylogenetic relationships within the Cercocebus-Mandrillus clade as indicated by craniodental morphology: Implications for evolutionary biogeography. American Journal of Physical Anthropology 158(2):227–241.
Disotell, T.R. 1994. Generic level relationships of the Papionini (Cercopithecoidea). American Journal of Physical Anthropology 94(1):47–57. https://doi.org/10.1002/ajpa.1330940105.
Fantini, L., M.D. Mudry, and M. Nieves. 2011. Genome size of two Cebus species (Primates: Platyrrhini) with a fertile hybrid and their quantitative genomic differences. Cytogenetic and Genome Research 135(1):33–41.
Fischer, S., B.P. Brunk, F. Chen, X. Gao, O.S. Harb, J.B. Iodice, Shanmugam, et al. 2011. Using OrthoMCL to assign proteins to OrthoMCL-DB groups or to cluster proteomes into new ortholog groups. Current Protocols in Bioinformatics 35:6.12.1–6.12.19.
Fleagle, J.G. 1999. Primate Adaptation and Evolution, Second Edition. San Diego, CA: Academic Press, pp. 199–200.
Fleagle, J.G., and W.S. McGraw.1989. Skeletal and dental morphology supports diphyletic origin of baboons and mandrills. Proceedings Of the National Academy of Sciences, USA 96(3):1157–1161.
Gebo, D.L. 2014. Primate Comparative Anatomy. Baltimore, MD: Johns Hopkins University Press, pp. 2–9.
Groves, C.P. 1970. The forgotten leaf-eaters and the phylogeny of the Colobinae. in Old World monkeys: evolution, systematics, and behavior (J.R. Napier and P.H. Napier, eds.). New York, NY: Academic Press, pp. 555–587.
Harris, E.E. 2000. Molecular systematics of the old world monkey tribe papionini: analysis of the total available genetic sequences. Journal of Human Evolution 38(2):235–256.
Hartwig-Scherer, S. 1993. Hybridisierung und artbildung bei den Meerkatzenartigen (Primates, Cercopithecoidea). In: Scherer, S., ed. Typen des Lebens. Berlin, Germany: Pascal-Verlag, pp. 245–257.
Hartwig-Scherer, S. 1998. Apes or ancestors? Interpretations of the hominid fossil record within evolutionary and basic type biology. In: Dembski, W.A., (ed.). Mere Creation. Downers Grove, IL: InterVarsity Press, pp. 212–235.
Kim, H.-S., and O. Takenaka. 1996. A comparison of TSPY genes from Y-chromosomal DNA of the great apes and humans: Sequence, evolution, and phylogeny. American Journal of Physical Anthropology 100(3):301–309.
Liedigk, R., C. Roos, M. Brameier, and D. Zinner, 2014. Mitogenomics of the Old World monkey tribe Papionini. BMC Evolutionary Biology 14(1):176, DOI:.
Lightner, J.K., and M. Cserhati. 2019. The uniqueness of humans is clearly demonstrated by the gene-content Statistical Baraminology Method. Creation Research Society Quarterly 55(3):132–141.
Line, P. 2013. Explaining robust humans. Journal of Creation 27(3):64–71.
Lubenow, M.L. 2004. Bones of contention: A creationist assessment of human fossils, Second Edition. Grand Rapids, Michigan: Baker Books.
Meyer, M., Q. Fu, A. Aximu-Petri, I. Glocke, B. Nickel, J.L. Arsuaga, I. Martínez, A. Gracia, J.M. de Castro, E. Carbonell, and S. Pääbo. 2014. A mitochondrial genome sequence of a hominin from Sima de los Huesos. Nature 505(7483):403–406.
Neusser, M., M. Münch, G. Anzenberger, and S. Müller. 2005. Investigation of marmoset hybrids (Cebuella pygmaea x Callithrix jacchus) and related Callitrichinae (Platyrrhini) by cross-species chromosome painting and comparative genomic hybridization. Cytogenetic and Genome Research 108(1-3):191–196.
O’Micks. J. 2016. Preliminary baraminological analysis of Homo naledi and its place within the human baramin. Journal of Creation Theology and Science Series B: Life Sciences 6:31–39.
O’Micks, J. 2017. Baraminology classification based on gene content similarity measurement. Creation Research Society Quarterly 54(1):27–37.
O’Micks, J. 2018a. Modern science catches up with Neanderthal man. Journal of Creation 30(1):38–42.
O’Micks, J. 2018b. Baraminic analysis of archaic and modern human genomes. Journal of Creation 32(3): 19–24.
Osterholz, M., L. Walter, and C. Roos. 2008. Phylogenetic position of the langur genera Semnopithecus and Trachypithecus among Asian colobines, and genus affiliations of their species groups. BMC Evolutionary Biology 8(1):58.
Park, M.A. 2009. Biological Anthropology, Sixth Edition. New York, NY: McGraw-Hill.
Perelman, P., W.E. Johnson, C. Roos, H.N. Seuanez, J.E. Horvath, M.A. Moreira, B.D. Kessing, J. Pontius, M. Roelke, Y. Rumpler, M.C. Schneider, A. Silva, S. O’Brien, J. Pecon-slattery. 2011. A molecular phylogeny of living primates. PLoS Genetics 7:e1001342.
Pugh, K.D., and C.C. Gilbert. 2018. Phylogenetic relationships of living and fossil African papionins: Combined evidence from morphology and molecules. Journal of Human Evolution 123:35–51.
Rahim, N.A., M. Gani, and M.T. Abdullah. 2014. Phylogenetic relationships of macaques (Cercopithecidae: Macaca) inferred from partial mitochondrial DNA (mtDNA) cytochrome oxidase II (COII) gene. Borneo Journal of Resource Science and Technology 4(1):42–51.
Rensch, T., D. Villar, J. Horvath, D.T. Odom, and P. Flicek. 2016. Mitochondrial heteroplasmy in vertebrates using ChIP-sequencing data. Genome Biology 17(1):139.
Rupe, C., and J. Sanford. 2017. Contested Bones. Waterloo, NY: FMS Publications.
Savanne, D. 2014. Denisovans menace evolution—A new chapter in the human origins debate. Journal of Creation 28(3):5–8.
Schneider, H., and I. Sampaio. 2015. The systematics and evolution of New World primates – A review. Molecular Phylogenetics and Evolution 82(Part B):348–357.
Stanke, M., and B. Morgenstern. 2005. AUGUSTUS: a web server for gene prediction in eukaryotes that allows user-defined constraints. Nucleic Acids Research 33(Web Server issue):W465-W467.
Stringer, C., and P. Andrews. 2011. The Complete World of Human Evolution, Second Edition. London, UK: Thames & Hudson Ltd.
Thompson, J.D., T.J. Gibson, and D.G. Higgins. 2002. Multiple sequence alignment using ClustalW and ClustalX. Current Protocols in Bioinformatics, Chapter 2.
Van Gelder, R.G. 1977. Mammalian hybrids and generic limits. American Museum Novitates 2635:1–25.
Whittaker, D.J., N. Ting, and D.J. Melnick. 2006. Molecular phylogenetic affinities of the simakobu monkey (Simias concolor). Molecular Phylogenetics and Evolution 39(3):887–892.
Wood, T.C. 2008. Animal and Plant Baramins. CORE (Center for Origins Research) Issues in Creation 3:1–258.
Wood, T.C. 2010. Baraminological analysis places Homo habilis, Homo rudolfensis, and Australopithecus sediba in the human holobaramin. Answers Research Journal 3:71-90.
Wood, T.C. 2016. An evaluation of Homo naledi and “Early” Homo from a young-age creationist perspective. Journal of Creation Theology and Science B: Life Sciences 6:14-30.