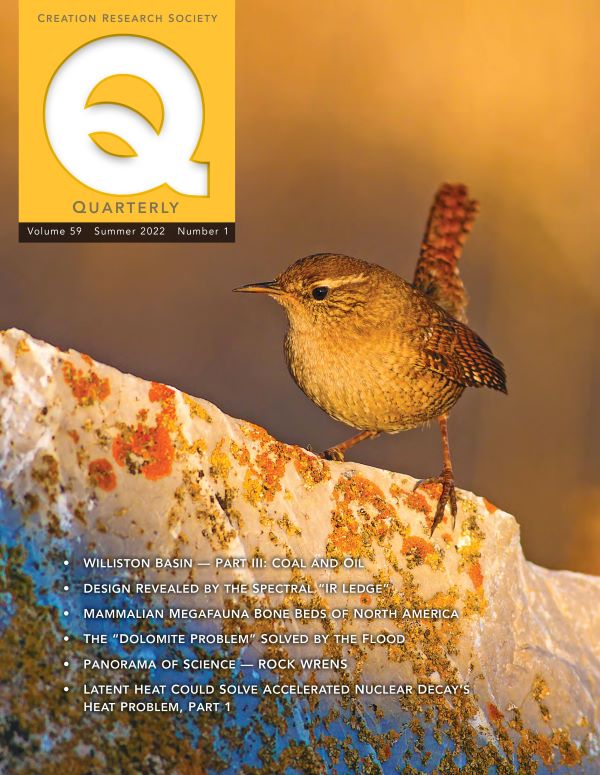
ABSTRACTS
by Jonathan K. Corrado
Remote sensing is a field designed to enable people to look beyond the range of human vision. Whether it is over the horizon or in a spectral range outside our perception, we search for information about our environment. Remote sensing data is enormously powerful and gives humans more data than can be discriminated directly via our senses. The extraction and analysis of this data reveals important, valuable information. It also implies specific design characteristics that only a designer could invoke. One of the incredible phenomena found through remote sensing is the illumination of the “IR Ledge” in vegetation. The IR Ledge or “red edge” is an essential and dramatic spectral feature found in remote sensing and refers to the region of dramatic change in reflectance of vegetation in the near infrared (NIR) range of the electromagnetic spectrum. This dramatic variation in reflectance facilitates photosynthesis and protects vegetation in the higher energy, longer wavelength region of the electromagnetic spectrum. This article contends that this reflectance phenomenon combined with the unique and precisely tuned blackbody radiation profile of the Sun centered on the visual spectrum displays characteristics that transcend coincidence, meets the definition of Complex Specified Information, and infers causation and, therefore, design.
Design Revealed by the Spectral “IR Ledge”
Introduction
“Astronomy may be revolutionized more than any other field of science by observations from above the atmosphere. Study of the planets, the Sun, the stars, and the rarified matter in space should all be profoundly influenced by measurements from balloons, rockets, probes and satellites.... In a new adventure of discovery no one can foretell what will be found, and it is probably safe to predict that the most important new discovery that will be made with flying telescopes will be quite unexpected and unforeseen.” – Lyman Spitzer, Jr.
Remote sensing is the process of detecting and monitoring the physical characteristics of an area by measuring its reflected and emitted radiation at a distance (typically from a satellite or aircraft) (Chuvieco, 2020). Said more practically, remote sensing is a field designed to enable people to look beyond the range of human vision. Whether it is over the horizon, beyond our limited range, or in a spectral range outside our perception, we are in search of information (Olsen, 2016). Special cameras collect remotely sensed images, which help researchers “sense” things about the Earth. First, cameras on satellites and airplanes take images of large areas on the Earth’s surface, allowing us to see much more than we can see when standing on the ground. Second, sonar systems on ships create ocean floor images without needing us to travel to the bottom of the ocean. Finally, cameras on satellites can be used to make images of ocean temperature changes.
Four specific uses of remotely sensed images of the Earth include mapping large forest fires from space to allow rangers to see a much larger area than from the ground. Second, scientific surveillance, e.g., tracking clouds to predict the weather, observing erupting volcanoes, or watching out for dust storms. Third, tracking city growth and changes in farmland or forests over several years or decades. Fourth, the discovery and mapping of the rugged topography of the ocean floor (e.g., huge mountain ranges, deep canyons, and the “magnetic striping” on the ocean floor) (Chuvieco, 2020).
Remote sensing data is enormously powerful and gives humans much more information than can be detected directly via our senses. The extraction and analysis of this data reveal important, valuable information for many different applications but implies specific design characteristics that only a designer could invoke. One such design element is a phenomenon called the “IR Ledge” used by image analysts to highlight vegetation in satellite imagery. The discussion of the IR Ledge and the design implied by this marvel is the substance of this paper, “for his invisible attributes, namely, his eternal power and divine nature, have been clearly perceived, ever since the creation of the world, in the things that have been made. So they are without excuse” (Romans 1:20).
Reflectance of Materials
Reflection illustrates the process whereby incident radiation recoils off the surface of a material in a single, anticipated direction. Reflection is produced by surfaces that are smooth relative to the wavelength of incident radiation. These smooth, mirror-like surfaces are called “specular reflectors” (Olsen, 2016). The reflectance of most substances varies with wavelength, which allows spectral imagers to distinguish different substances. Figure 1 (spectral image) illustrates reflective spectra. Spectra are the fingerprints of elements, derived from their fundamental atomic characteristics.
[FIG. 1]
Figure 1. Spectral reflectance curve for an oak leaf (Kokaly et al., 2017).
Knowledge of spectral signatures is essential in the understanding and interpretation of a remotely sensed image. Wavelength-dependent absorptions distinguish different substances, and these images of reflected solar energy are known as “spectral signatures.” The property used to quantify these spectral signatures is called spectral reflectance. This reflection is a ratio of the reflected energy to incident energy as a function of wavelength (Kruse, 1999). The graph of the spectral reflectance of an object as a function of wavelength is called the “spectral reflectance curve” (Lillesand and Kiefer, 1999).
The arrangement of the spectral reflectance curves is essential in establishing the wavelength region(s) in which remote sensing data is obtained, as the spectral reflectance curves provide comprehension of the spectral characteristics of an object (Lillesand and Kiefer, 1999). Spectral signatures obtained from multispectral images are isolated compared to the adjoining signatures obtained from hyperspectral images. Adjoining spectral signatures allow for detailed analysis through the recognition of surface materials and their proportion, as well as deductions of biological and chemical processes (Lillesand and Kiefer, 1999). As displayed in Figure 1, the reflectance curve for an oak leaf illustrates the reflectance curve unique to that leaf.
In green vegetation, the plant’s coloration regulates the valleys in the visible portion of the spectrum. For example, chlorophyll absorbs sharply in the blue (450 nm) and red (670 nm) sections of the electromagnetic spectrum, otherwise known as the “chlorophyll absorption bands.” Chlorophyll is the primary photosynthetic pigment in green plants (Lillesand and Kiefer, 1999). Thus, the human eye perceives healthy vegetation as green due to the strong absorption of the red and blue wavelengths and the reflection of the green wavelengths. When the plant encounters stress that hinders normal growth and chlorophyll production, less adsorption occurs in the red and blue regions and the amount of reflection in the red waveband increases (Lillesand and Kiefer, 1999).
The spectral reflectance signature illustrates a dramatic increase in the reflection for healthy vegetation at around 700 nm. In the near-infrared (NIR) range between 700 and 1300 nm, a plant leaf will typically reflect between 40 to 50%; the rest is transmitted, with only about 5% being adsorbed. Structural variability in leaves in this range allows one to differentiate between species, even though they might look the same in the visible region (Lillesand and Kiefer, 1999).
The “IR Ledge”
The “IR Ledge” or “red edge” is an important and dramatic spectral feature found in remote sensing, as can be seen around 700 nm on a spectral image of green vegetation. The IR ledge refers to the region of dramatic change in vegetation reflectance in the near-infrared range. This striking wavelength reflectance rise makes vegetation appear bright in the infrared, as displayed in the false-color images in Figures 2 and 3. As previously discussed, the leaf pigments, cell structure and water content all impact the spectral reflectance of vegetation (Carter, 1991). In the visible bands, the reflectance is relatively low as the leaf pigments absorb the most light. For healthy vegetation, the reflectance is much higher in the near-infrared region than in the visible region due to the cellular structure of the leaves. Therefore, healthy vegetation can be easily identified by the high near-infrared reflectance and generally low visible reflectance.
[FIG. 2]
Figure 2. Natural- and false-color images from NASA’s MESSENGER mission to Mercury showing plant-covered land from the Amazon rainforest to the North American forests (V1Media/Earth Imaging Journal, 2014).
[FIG. 3]
Figure 3. QuickBird near-infrared satellite image of Nigeria, Africa (Satellite Imaging Corporation).
Live green plants absorb solar radiation in the photosynthetically active radiation (PAR) spectral region, which they use as a source of energy in the process of photosynthesis. Leaf cells also re-emit solar radiation in the near-infrared spectral region (which carries approximately half of the total incoming solar energy) because the photon energy at wavelengths longer than about 700 nm is too small to synthesise organic molecules. Strong absorption at these wavelengths would only result in overheating the plant and possibly damaging the tissues. Hence, live green plants appear relatively dark in the PAR and relatively bright in the near infrared (Gates, 1980). By contrast, clouds and snow tend to be relatively bright in the red (as well as other visible wavelengths) and quite dark in the near infrared. As previously discussed, pigment in plant leaves, chlorophyll, strongly absorbs visible light (from 400 to 700 nm) for use in photosynthesis. The cell structure of the leaves, on the other hand, strongly reflects near-infrared light (from 700 to 1100 nm). The more leaves a plant has, the more these wavelengths of light are affected.
The Design of the IR Ledge
Blackbody radiation of the Sun
When an object is heated to a high temperature, it emits light that is more representative of the object’s temperature than of its composition. When an object has this characteristic, it is called a “blackbody radiator.” Blackbody radiation is the thermal electromagnetic radiation within or surrounding a body in thermodynamic equilibrium with its environment, emitted by a blackbody (an idealized opaque, non-reflective body) (Stewart and Johnson, 2017). Typically, light emitted by these objects has similar characteristics in that the blackbody produces a continuous spectrum (over a wide range of wavelengths). The wavelength of the maximum radiation intensity varies inversely with the temperature. The total energy emitted varies as the fourth power of the temperature, and the power at a given temperature varies inversely with the fifth power of the wavelength (Stewart and Johnson, 2017).
These characteristics result in emission spectra like that shown in Figure 4.
[FIG. 4]
Figure 4. Emission spectra of blackbodies at various temperatures (Tanaka et al., 2010).
As the temperature increases, the peak of the emitted blackbody radiation curve moves to higher intensities and shorter wavelengths (up and to the left on Figure 4). The 5250 °C (9482 °F) line in the figure corresponds roughly to the temperature of the Sun’s photosphere. Although planets and stars are neither in thermal equilibrium with their surroundings nor perfect blackbodies, researchers use blackbody radiation as a first approximation for the energy planets and stars emit (Stewart and Johnson, 2017).
Anthropic implications
As depicted in Figure 4, the peak of the 5250 °C curve centers in the visible spectrum and the most (and highest) energy is emitted within this visual band. As the Sun represents the 5250°C curve, it would effectively need to remain at a stagnate temperature (given the distance from the Sun to Earth), or life of any kind on Earth would be impossible as implied by the “Anthropic Cosmological Principle” (Borrow and Tipler, 1988). If the surface temperature of the Sun increased, two main consequences would result. First, the total energy radiated from the Sun would increase (and so Earth would get hotter). Second, the Sun’s light wavelength would decrease (the Sun would become bluer). The first would cause severe climate change, and the latter would result in much higher levels of ultraviolet radiation reaching Earth. If this transition were to happen suddenly, life on Earth would quickly go extinct. If it happened gradually, some microbial life-forms might survive in the new environment, but plant, animal, and human life would cease to survive (Borrow and Tipler, 1988).
So, with the understanding that the Sun’s temperature has to remain relatively stagnant for life on Earth (as we know it) to remain viable, with the blackbody spectrum remaining constant, it can be logically inferred that the placement of the IR Ledge should be tied to the Sun’s blackbody spectrum. As displayed in Figure 5, an overlay of the Sun’s blackbody radiation profile on top of a reflectance curve of vegetation clearly shows this tie. From the figure, most of the Sun’s energy is emitted within the wavelength range of the visible spectrum, which is precisely the energy absorbed by plant life for the photosynthesis process. Outside the visual spectrum, the Sun’s blackbody energy declines rapidly as the plant’s reflectance profile increases promptly to repel the energy at wavelengths that would harm the plant. This phenomenon indicates complexity, sophistication, and purpose—as if it were designed by a designer.
[FIG. 5]
Figure 5. Plot of the Sun’s blackbody radiation overlaid on a reflectance curve for an oak leaf (Coddington et al., 2015; Kokaly et al., 2017).
The irresolute evolution of photosynthesis
Photosynthesis is a process used by plants and other organisms to convert light energy into chemical energy that, through cellular respiration, can later be released to fuel the organism's metabolic activities. This chemical energy is stored in carbohydrate molecules, such as sugars and starches, which are synthesized from carbon dioxide and water. There are two types of photosythesis. The first is anoxygenic photosynthesis that uses molecules other than water to drive the process and does not produce oxygen as a byproduct. The second is oxygenic photosynthesis, which separates water into hydrogen and oxygen to drive photosynthesis, and releases oxygen as a byproduct. Oxygenic photosynthesis is the most common and familiar, found in algae, plants, and in some bacteria. Oxygenic photosynthesis is a fundamental process that sustains human and animal life (Bryant and Frigaard, 2006).
For decades, biological evolutionary theory contented that anoxygenic photosynthesis significantly predated oxygenic photosynthesis on the order of a billion years. However, recent research discovered unique structures in bacteria, specifically Heliobacterium modesticaldum, demonstrating that oxygenic photosynthesis was occurring a billion years earlier than commonly thought. By investigating H. modesticaldum, research found fully functional photosynthesis was already in place, therefore, one kind of photosynthesis could not have evolved into the other (Cardona, 2019). This discovery leans in favor of intelligent design versus adaptive response over time in that this recent finding points more firmly to the fact that the molecules and processes that enable photosynthesis were purposely formed for that specific function.
Further, the formation of these complex, photosynthetic molecules comprises a set of enzymes that must act in a specific sequence. Per the Granick Hypothesis, these enzymes evolved arbitrarily and the sequence of steps in the synthesis represents the progression of steps in the historical evolution of the process. But the probability of this natural selection bringing about the right enzymes in the right sequence at the right time to produce the end product surpasses reason and transcends incongruity. The Calvin Cycle, a process that plants and algae use to turn carbon dioxide from the air into sugar, necessitates eleven different enzymes, all of which are uniquely coded within the DNA and directed specifically to the chloroplast, a component that contains chlorophyll and in which photosynthesis takes place. For the Calvin Cycle to function correctly, all enzymes must be present (Taiz and Zeiger, 1998).
It thwarts logic to presume the irreducible complexity of photosynthetic systems would have resulted from arbitrary means and randomness. Rather, the specific arrangement and complexity apparent in photosynthesis, a process scientists have merely scratched the surface in comprehending, more comfortably and logically aligns with design. Moreover, the concept of an unevolved photosynthetic process would also suggest against an evolving, shifting electromagnetic spectrum emitted from the Sun. The solar spectrum emitted and the photosynthetic receptors need to be in lockstep for the process to function and life on Earth to exist. As this suggests, ‘success tapping’ into the electromagnetic wavelengths arriving from the Sun would not be necessary, thus disputing the idea that if a different spectrum reached the surface of the Earth, maybe a different chemical process would have been utilized for photosythesis.
Specified complexity
‘The concept of specified complexity’ asserts that when something exhibits specified complexity (i.e., the item or phenomenon is both complex and specified, simultaneously), one can infer an intelligent cause (i.e., that it was designed) rather than being the result of natural processes (Dembski, 1999). Any object or event with an extremely low probability of occurring by chance that matches a discernable pattern displays specified complexity. According to contemporary design theory, the presence of highly specified complexity is an indicator of an intelligent cause. Although the theory of specified complexity derived by William Dembski stemmed from his study of biology, this idea can be expanded to other fields of science and occurrences in nature.
Complex Specified Information (CSI), the qualitative and quantitative measure of specified complexity, is a reliable indicator of design because its recognition corresponds with how researchers identify intelligent causality. In general, to distinguish intelligent causality, one must establish that a choice from a range of contending possibilities was definitely actualized, determine which possibilities were excluded, and then identify the particular possibility that was actualized. Besides, the excluded competing possibilities must be tangible possibilities, suitably numerous, so that identifying the possibility that was actualized cannot be attributed to chance. Regarding probability, this means that the identified possibility is highly improbable. Regarding complexity, this means that the identified possibility is highly complex. All the components in the general method for identifying intelligent causation (i.e., actualization → exclusion → specification) find an equivalent in CSI (Dembski, 1997).
As displayed in Figure 5, most of the Sun’s energy is emitted and peaks in the visual range of the electromagnetic spectrum, thus making life on Earth possible. In tandem, the nested vegetation spectral reflectance curve indicates high absorption within the visual spectrum. This absorption facilitates photosynthesis and rapidly transitions to high reflection values outside the visual band to protect the plant from the more harmful longer wavelengths. Based on the previous discussion of the irresolute evolution of photosynthesis, it can be assumed that the probability of the Sun emitting a majority of its energy in the spectral range to be a low probability. It can also be assumed that the probability of the vegetation’s spectral reflectance profile indicating high absorption in the visual range and a rapid transition to the higher reflectance right outside the visual range also to be low. Collectively, the composite probability of these two independent probabilities would yield an even lower probability. This construction reduces the probability of this condition occurring by chance and infers that this is a “complex” relationship as defined in the concept of specified complexity.
The distinction between specified and unspecified information can be defined as follows: “the actualization of a possibility (i.e., information) is specified if independently of the possibility’s actualization, the possibility is identifiable by means of a pattern. If not, then the information is unspecified” (Dembski, 1997). Note that this definition implies an asymmetry between specified and unspecified information. Specified information cannot become unspecified information, though unspecified information may transform into specified information. Unspecified information can become specified as our background knowledge increases. In the context of Figure 5, independently, the blackbody curve and the vegetation reflectance curve can be deemed “specified” per the above definition concerning the visual spectrum and their characteristics within this wavelength range. Taken collectively, logically, the relationship between the two curves can be inferred as a “specified” relationship.
As postulated, the IR Ledge combined with the precisely arranged blackbody radiation profile of the Sun centered on the visual spectrum presented in Figure 5 is determined to be both “complex” and “specified.” Therefore the IR Ledge can be labeled “Complex Specified Information,” which, per the above discussion qualitatively, infers a high likelihood of causation and thus design.
Conclusion
“For by him all things were created, in heaven and on Earth, visible and invisible, whether thrones or dominions or rulers or authorities—all things were created through him and for him. And he is before all things, and in him all things hold together” (Colossians 1:16-17).
Evidence of design is continuously overwhelming our senses. It does not take much rational thought to conclude that our material existence resulted from design in contrast to accidental, evolutionary causes. From the macro to the micro, the elements of our material existence are finely balanced and operate with intricate, shared precision. Some of the most remarkable findings of modern physics and cosmology, especially the indications that our particular universe and its laws seem remarkably tailored to sustain life, support this point of view.
Via remote sensing in the near infrared, one of these interesting yet critical design elements appears in the IR Ledge. This appearance combined with the unique and specially tuned blackbody radiation profile of the Sun centered on the visual spectrum displays characteristics that transcend coincidence, meet the definition of CSI, and thus radiate the inference of design “for from him and through him and to him are all things. To him be glory forever, Amen” (Romans 11:36).
References
Borrow, J., and F. Tipler. 1988. The Anthropic Cosmological Principle. Oxford University Press, New York, NY.
Bryant, D.A., and N.U. Frigaard. 2006. Prokaryotic photosynthesis and phototrophy illuminated. Trends in Microbiology 14(11): 488–496. doi:10.1016/j.tim.2006.09.001. PMID 16997562.
Cardona, T. 2019. Thinking twice about the evolution of photosynthesis. Open Biology 9(3): 180246. http://doi.org/10.1098/rsob.180246 .
Carter, G. 1991. Primary and secondary effects of water content on the spectral reflectance of Leaves. American Journal of Botany 78(7): 916-924. https://doi.org/10.1002/j.1537-2197.1991.tb14495.x .
Chuvieco, E. 2020. Fundamentals of Satellite Remote Sensing: An Environmental Approach, third edition. CRC Press/Taylor & Francis Group, Boca Raton, FL.
Coddington, O., J.L. Lean, D. Lindholm, P. Pilewskie, M. Snow, and NOAA CDR Program. 2015. NOAA climate data record (CDR) of solar spectral irradiance (SSI), NRLSSI version 2. NOAA National Centers for Environmental Information. https://doi.org/10.7289/V51J97P6 (accessed April 15, 2021).
Dembski, W. 1997. Intelligent design as a theory of information. Access Research
Network.
Dembski, W. 1999. Intelligent Design: The Bridge Between Science and Theology. Intervarsity Press, Downers Grove, IL.
[See V1 Media (the copyright source of this article) below.]
Gates, D.M. 1980. Biophysical Ecology. Springer-Verlag, New York, NY.
Kokaly, R.F., R.N. Clark, G.A. Swayze, K.E. Livo, T.M. Hoefen, N.C. Pearson, R.A. Wise, W.M. Benzel, H.A. Lowers, R.L. Driscoll, and A.J. Klein. 2017. USGS Spectral Library Version 7: U.S. Geological Survey Data Series 1035. https://doi.org/10.3133/ds1035.
Kruse, F.A. 1999. Visible-Infrared Sensors and Case Studies. In Remote Sensing for the Earth Sciences: Manual of Remote Sensing, third edition. A.N. Renz (editor). Volume 3, pp. 567-611. John Wiley & Sons, New York, NY.
Lillesand, T., and R. Kiefer. 1999. Remote Sensing and Image Interpretation. John Wiley & Sons, Inc., Hoboken, NJ.
Olsen, R. 2016. Remote Sensing from Air and Space, second edition. Spie Press, Bellingham, WA.
Satellite Imaging Corporation. 2004. QuickBird Near Infrared Satellite Image Nigeria, Africa. Satellite Imaging Corporation; image acquired February 3, 2004. https://www.satimagingcorp.com/gallery/quickbird/quickbird-oil-and-gas-near-infrared/ (accessed April 15, 2021).
Stewart, S., and R. Johnson. 2017. Blackbody Radiation: A History of Thermal Radiation Computational Aids and Numerical Methods. CRC Press/Taylor & Francis Group, Boca Raton, FL.
Taiz, L., and E. Zeiger. 1998. Plant Physiology, second edition. Sinauer Associates, Inc., Massachusetts, MA.
Tanaka, Y., et al. 2010. Long-lasting muscle thinning induced by infrared irradiation specialized with wavelength and contact cooling: A preliminary report. ePlasty 10:e40.
V1 Media. 2014. How to interpret a false-color satellite image. Earth Imaging Journal, April 11. https://eijournal.com/print/articles/how-to-interpret-a-false-color-satellite-image (accessed April 15, 2021).
Captions
Figure 1. Spectral reflectance curve for an oak leaf.
Figure 2. Natural- and false-color images from NASA’s MESSENGER mission to Mercury showing plant-covered land from the Amazon rainforest to the North American forests.
Figure 3. QuickBird near-infrared satellite image of Nigeria, Africa.
Figure 4. Emission spectra of blackbodies at various temperatures.
Figure 5. Plot of the Sun’s blackbody radiation overlaid on a reflectance curve for an oak leaf.