ABSTRACTS
Mark H. Armitage and George F. Howe
THE ULTRASTRUCTURE OF LICHEN CELLS SUPPORTS CREATION, NOT MACROEVOLUTION
A Photo Essay and Literature Review—Part 1 (A Van Andel Creation Research Center Report)Summer 2007, CRSQ Vol 44 No 1 pp 40-53
INTRODUCTION
Lichens are a life-form composed of a fungus called the “mycobiont,” growing in close union with some alga or blue-green bacterium known as the “phycobiont” (Howe and Armitage, 2002). Some workers consider this to be a symbiotic union, while others see it as a mild form of parasitism. Lichen pigments probably play many important physiological roles, while their brilliant colors provide considerable aesthetic enjoyment (Howe and Armitage, 2003). The versatile array of lichen asexual reproductive bodies and other features of the lichen upper surface have been illustrated by using scanning electron photomicrography (Armitage and Howe, 2004). Lichen algae and fungi are woven together forming “tissues” that resemble the tissues of unrelated “higher plants” in a generalized fashion (Armitage and Howe, 2006). In this two-part article (our fifth lichen paper) we show that the cellular ultrastructure of lichen provides evidence favoring design and direct creation. Our hope here, as with the other lichen papers, is that the readers’ knowledge of the Creator and their esteem for His handiwork will be enhanced by the study of lichens.
METHODS
The methods we used in sectioning the lichens and securing the electron photomicrographs are the same as those discussed in previous papers (Armitage and Howe, 2004; 2006).
THE ULTRASTRUCTURE OF LICHEN ALGAE AND FUNGILICHEN ALGAE AND FUNGI Lichens Have Tissues
The cells seen in our Transmission Electron Microscope (TEM) photographs are located either in the algal zone or in the medulla tissue of various lichens (Figure 1). Underneath their protective upper cortex tissue, lichens typically possess an “algal layer,” which also contains the lichen fungus. The fungus filaments are called “hyphae” and they make up a “mycelium” network. They are usually subdivided by numerous cross walls into individual sections, which are considered to be “cells,” even though the fungus in lichen is often one interconnected body.
The fungus hyphae are in direct contact with the cells of algae (see Figure l). The algae are equipped to store the energy of light through photosynthesis, and because of the close contact with mycobiont cells, they can supply food to the whole lichen system. Figures 2-8, together with Figure 12, are higher magnification photomicrographs of phycobiont and mycobiont cells found in the algal layer of certain lichens, which grow at the Creation Research Society's Van Andel Creation Research Center (Chino Valley, Arizona). Figures 9-11 are pictures of mycobiont cells from the medulla layer of those lichens.
Trebouxia sp. is the Main Lichen Alga. Figure 2 shows cells of the green alga Trebouxia sp. found together with fungal cells in the lichen Caloplaca sp. Sections of the Trebouxia phycobiont also can be seen in Figures 3-8 and Figure 12. Trebouxia is the algal genus present in the majority of lichen species worldwide. Ahmadjian (2001) has recognized about 30 different species in the algal genus Trebouxia.
Figure 1. A brightfield photomicrograph of a cross section of a typical foliose lichen Xanthoparmelia sp. with its tissue zones labeled. The tissues are: upper cortex (1), algal layer (2), medulla (3), and lower cortex (4). Area (5) appears to be a developing reproductive structure called an apothecium. A Trebouxia cell in the algal layer is labeled “t,” while item “f” is a fungus hypha in the medulla. Lichens are thought to be very distant taxonomically from flowering plants, yet many features found in the tissues of plant leaves are duplicated here in a very generalized fashion (Armitage and Howe, 2004; 2006). A mycobiont produces a complex thallus like this only when it grows with its phycobiont. The same fungus by itself builds no such edifice. This suggests intelligent design. The scale bar is 60 micrometers, and the magnification is 2,000X.
Figure 2. A transmission electron microscope (TEM) photograph of Trebouxia sp., a green alga present in many different lichens, and two accompanying fungal cells from the algal zone of the lichen Caloplaca sp. This figure, and all those which follow, are TEM photographs. The numbered visible items here are a cell of the phycobiont Trebouxia sp.: chloroplast (6), pyrenoid inside chloroplast (7), pyrenoglobuli (8), thylacoid membranes inside the chloroplast (9), cytoplasm (10), mitochondria (11), cytoplasmic membrane (usually called the plasmalemma) (12), storage region just outside the plasma lemma (13), cell wall (14), cell of the fungus in the Caloplaca lichen (15), nucleus (16), nucleolus (17), and an artifact (18). Dark dots like these on other figures are artifacts as well. The magnification is 11,000X, and the scale bar is 4 micrometers.
Figure 3a. The chloroplast of a Trebouxia algal cell from the algal zone of Caloplaca sp. The chloroplast of Trebouxia is deeply cleft, forming lobes along its margin (19). Thylacoid membranes of the chloroplast are arranged into grana-like stacks (20), and algal mitochondria (11) are visible in the surrounding cytoplasm (10). The magnification is 14,000X, and the scale bar is 2 micrometers.
Figure 3b. Another chloroplast of Trebouxia sp. from the alga Caloplaca. The size bar is 1 micrometers. The grana-like stacks of membranes in the chloroplast (20) can be seen here in several locations as well as the cytoplasm (10) and the pyrenoglobuli (8).
Figure 4. A cell of the phycobiont Trebouxia sp. from the algal zone of the lichen Candelariella sp. [Note: Candelariella is the same yellow orange lichen that we mistakenly called Candelilaria in our previous articles (Armitage and Howe 2004; 2006), and correctly identified in Howe and Armitage, 2003]. In addition to the storage layer (13) located just outside the plasmalemma (12), many white storage areas can be observed (21) at the outer edge of the cytoplasm. Pyrenoglobuli (8), which store lipids, are also present in the pyrenoid of the chloroplast. At some locations several pyrenoglobuli are arranged in an orderly line because of their attachment to the thylacoid membranes of the pyrenoid. Numbers for other subcellular particles are as on the previous figures, (6) chloroplast, (7) pyrenoid, and (14) cell wall. Two mycobiont cells (15) are closely appressed to the alga. The three different storage systems seen here enable lichens to thrive despite long periods of unfavorable climate. The magnification is 10,000X, and the scale bar is 6 micrometers.
Photosynthesis and Storage Occur in the Chloroplasts of Trebouxia
Trebouxia chloroplasts are visible in Figures 2–8, where they are labeled “6.” Trebouxia has a single, gigantic chloroplast surrounded by a narrow layer of cytoplasm (see Peveling, 1973, p. 149). Inside and weaving throughout the chloroplast are many membranes known as thylacoids. In various algae, these thylacoids are stacked one upon another, forming disk-shaped complexes called grana (singular granum). Fisher and Lang (1971b) noted that in lichen algae the chloroplast membranes are often stacked this way. But Peveling (1973) reported that the usual stacking of thylacoids was absent in most species of Trebouxia. Nonetheless, she did find grana present in the Trebouxia phycobionts of Caloplaca. The Trebouxia cell from the lichen Caloplaca (seen as number 20 in our Figures 3a and 3b) likewise has grana-like stacks of thylacoid membranes.
Attached to the many membranes of the chloroplast is an incomprehensibly intricate assemblage of enzymes, carriers, and chlorophyll molecules, by which solar energy is transduced effectively, becoming the chemical bond energy of sugars and other photosynthates (organic molecules produced in photosynthesis). While converting carbon dioxide into sugar during photosynthesis, lichens likewise release generous quantities of oxygen gas into the atmosphere as a by-product. Though not to be reviewed in the paper, the complex details of photosynthesis provide firm evidence favoring intelligent design. Some lichens are large, but most are small. Because of their widespread global distribution, lichens yield a substantial share of the world’s photosynthate. This is reminiscent of God’s challenging words in Zechariah 4:10 to the Israelites as they were rebuilding their temple: “Who hath despised the day of small things?” (KJV).
Figure 5. A Trebouxia sp. phycobiont cell surrounded by many fungal cells (15) in the algal zone of Candelariella sp. In this one section, at least 10 fungal cells are seen in close contact with the one algal cell, upper center, where one whole cell and parts of two others are visible (6). Nuclei with nucleoli are evident in various algal and fungal cells (16). Nucleoli store and transport messenger RNA molecules, which in turn encode for the production of proteins. The magnification is 8,000 X, and the scale bar is 10 micrometers.
Within the chloroplast of Trebouxia is a large central region called the pyrenoid (see number “7” on Figures 2, 4, 5, and 8). Pyrenoids contain proteins and lipids, and they are likewise centers for starch synthesis. The pyrenoid is a region where membranes are present, but are not as numerous as elsewhere in the chloroplast (see Fisher and Lang, 1971b, p. 156).
Pyrenoids occur in many other algae besides Trebouxia and even in the chloroplasts of Anthoceros, a diminutive, mosslike plant called the “hornwort” (Vaughn et al., 1990). Hornworts are placed in the Plantae, the kingdom of “true plants,” while green algae such as Trebouxia are assigned to an entirely different kingdom, the Prokaryotae, which includes various divisions of algae. The presence of pyrenoids in these two widely separated taxa (hornworts and green algae) has not been accounted for by evolution theorists.
In the pyrenoids of various algae and the hornwort plants is found an important enzyme directly involved in the photosynthetic fixation of carbon dioxide: ribulose-l,5-biphosphate carboxylase/oxygenase, known as Rubisco (Borkhsenious et al., 1998). Rubisco also occurs in certain hornworts that have no pyrenoid and in higher plants, which likewise do not possess pyrenoids. The pyrenoids contained in Trebouxia algae and in hornwort plants also possess an important carbon-concentrating mechanism by which the carbon dioxide concentration in the cell is enhanced and the production of photosynthates is thereby increased (Smith and Griffiths, 1996).
These and other striking similarities between organisms that are widely separated in taxonomy (green algae and hornworts) deserve further attention in origins discussions. Some evolution theorists hold that higher plants developed through a green algal stage and then to a liverwort-like ancestral form. The hornworts are not considered to be in the direct line of descent proposed from green algae to higher plants but are thought to be a side branch. And yet it is the hornworts, not the liverworts, that possess pyrenoids found in the supposed green algal “ancestors.” This puzzling fact presents difficulty for proposed schemes for the evolutionary descent of higher plants. It would be more in line with evolution theories if the chloroplasts of liverworts, not hornworts, possessed a pyrenoid.
Figure 6. Three Trebouxia phycobiont cells in close contact with four fungal cells. Thylacoid membranes (20) can be seen here in the lichen Caloplaca sp. Some of the features labeled and identified in previous figures are numbered here as well. Magnification is 11,000 X, and the scale bar is 5 micrometers.
Figure 7. Trebouxia phycobiont cell from the algal zone of the lichen Xanthoparmelia sp. The following features can be easily distinguished: white storage regions in the cytoplasm (21), storage layer between the plasmalemma and the cell wall (13), a lobe of the large chloroplast (19), nucleus and nucleolus of the Trebouxia cell (16), cell wall of the mycobiont (14m), cell wall of the phycobiont (14p), and the stacks of thylacoid membranes (20). Note above, center, the relatively thin cell walls the mycobiont hypha has here in the algal zone of this lichen and compare to the thick walls it produced in the medulla tissue of the same lichen species—Xanthoparmelia (see caption of Figure 10). These differences enable the fungus to receive photosynthate from nearby algal cells, whereas in the medulla the thicker walls supply support (see Armitage and Howe, 2006, pp. 258, 261). The magnification is 12,000X, and the scale bar is 4 micrometers.
Figure 8. A cell of the green alga Trebouxia from the algal zone of the lichen Candelariella sp. Pyrenoglobuli (8) are visible, attached in an orderly fashion to the thylacoid membranes of the pyrenoid (7). Pyrenoglobuli contain energy-rich lipids and may perform other important tasks, such as supplying water. Magnification is 11,000 X, and scale bar is 5 micrometers.
What Are the Pyrenoglobuli?
The numerous small dark (electrondense) circular objects visible in the pyrenoid of Trebouxia cells are called pyrenoglobuli (singular pyrenoglobule) and are labeled with a number “8” on Figures 2, 3b, 4, and 8. Another name for them historically was osmophilic globules. Several tests indicated pyrenoglobuli are lipid-rich storage deposits (Jacobs and Ahmadjian, 1969; Ahmadjian, 2001; Fisher and Lang, 1971a). These lipids may “help lichens survive extreme drying” (Egan, 2006).
The pyrenoglobuli are associated with and adjacent to the thylacoid membranes (Fisher and Lang, 1971a), along which they are positioned in a regular pattern, opposite one another (Fisher and Lang, 1971b; Peveling 1976; Brown and Wilson, 1968). The orderly arrangement of pyrenoglobuli, which is caused by this patterned attachment to thylacoids, can be seen labeled “8” at certain locations in our Figure 8.
Pyrenoglobuli are present in the chloroplasts of algae from “most algal classes” (Peveling, 1976, p. 18). Pyrenoglobuli are not limited to Trebouxia and have been observed in other photobionts and in free-living algae, as Ahmadjian (1993) pointed out. Ahmadjian (1993) also presented the following list of organisms that have pyrenoids with pyrenoglobuli: the alga Stichococcus (both in lichens and living free), zoochlorellae (algae living inside marine animal cells), some species of the alga Chlorella, some marine algae, and the hornwort plants.
Figure 9. A cell of the mycobiont of Candelariella sp. Invaginations of the plasmalemma (22) greatly increase the membrane surface area, thus fostering added contact necessary for various functions. In the center of the cell are three mitochondria (11). Folds of the mitochondrial inner membrane (23) contain a host of enzymes required for the respiratory synthesis of ATP, which is sometimes called the high energy “currency” of the cell. Several other items labeled in previous figures are numbered here as well. Magnification is at 14,000X, and the scale bar is 2 micrometers.
Plastoglobuli, objects that are similar to pyrenoglobuli, have been observed in the stroma (spaces between the grana) of chloroplasts in angiosperms (flowering plants) such as onions, spinach, and Tradescantia (Lichtenthaler, 1968). Pyrenoglobuli and these very similar plastoglobuli are widespread in phycobionts, free-living algae, and plants. Although plastoglobuli were at first assumed to be unique evolutionary productions found only in phycobionts, this evolution-related error was corrected.
There is no absolute certainty concerning the functions of pyrenoglobuli in lichens, but they probably serve as storage centers for lipids and they likely yield supplies of energy and water for the mycobionts (Peveling, 1973; Brown and Wilson, 1968). Secondary carotinoid pigments have been found stored in the pyrenoglobuli (Tschermak-Woess, 1989). Based on microchemical and photographic data, several other functions have been proposed for pyrenoglobuli, including “protection of algae from radiation” (Jacobs and Ahmadjian, 1969, p. 238) and helping in the development of lamellae (thylacoids) for the chloroplasts (Brown and Wilson, 1968).
The Synthesis of Starch also Occurs in Trebouxia Chloroplasts
Starch is a polymer containing many interconnected glucose sugar units. In algae, the pyrenoid is the center for the synthesis of starch. In the pyrenoids of Trebouxia, however, starch is produced normally only when the lichen is living in moist conditions. By way of contrast, the synthesis of lipids in the pyrenoglobuli of Trebouxia occurs under both moist and dry circumstances (see Jacobs and Ahmadjian, 1969, p. 238).When starch is manufactured in the chloroplast, it collects as visible droplets, which move to the edge of the chloroplast and are found in the stroma. Such white starch reserves have been photographed by other workers but are not seen in our figures.
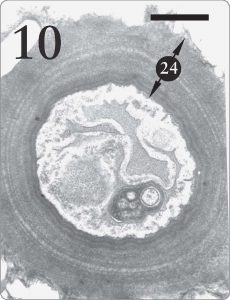
Is Starch Storage an “Ancestral Trait” in Trebouxia?
Jacobs and Ahmadjian (1969) postulated that Trebouxia evolved from a different green algal ancestor. Accordingly, they speculated that Trebouxia’s ability to store starch was an “ancestral” trait, which persisted after having been somewhat replaced by the lipid storage mechanism in the pyrenoglobuli (Jacobs and Ahmadjian, 1969, p. 238). But one might theorize instead that starch and lipid storage systems are simply two different alternatives, each designed to enable Trebouxia to cache photosynthate under diverse ecological regimes. In fact, Jacobs and Ahmadjian (1969) penned a very “design friendly” remark along that theme by stating that: a better way of viewing this is that lipids present in the pyrenoglobuli represent a type of storage product that is more suitable to the lichen symbiosis. In their natural habitats, lichens have only a few hours during the day with an adequate water balance for metabolism…Lipids would represent a product which would give the greatest amount of energy in the shortest possible time. (p. 238) Although they embraced a different origins model than creation, their statement here would fit well with a design scenario wherein the Creator endowed Trebouxia with starch storage for moist circumstances and a lipidpyrenoglobuli program for periods of low moisture. Starch synthesis is thus a useful secondary system and not an “ancestral trait.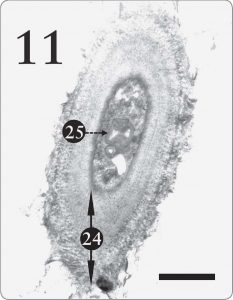
Sucrose Synthesis—Is it Ancestral or Alternate to Ribitol Production?
In its normal growth as a lichen photobiont, the alga Trebouxia manufactures the sugar-alcohol called ribitol, which moves into the adjacent fungal cells (Jacobs and Ahmadjian, 1969). When cultured axenically (by themselves), however, Trebouxia cells yield sucrose, not ribitol, as a photosynthate. The fabrication of sucrose or ribitol may be design alternatives. It is merely an evolutionary assumption that the ability to synthesize ribitol arose after the origin of sucrose production or that sucrose synthesis is somehow an “evolutionary vestige.”Lichen Algae and Fungi Possess Cytoplasm
The cytoplasm seen in both the alga and the fungus is not an unstructured matrix but “manifests internal order and numerous particulate bodies” (Howe, 1966, p. 106). It is designated as number “10” in Figure 2, 3b, and various other figures. Crossing the cytoplasm transversely is a finely structured, interweaving network of membranes, known as the endoplasmic reticulum, which holds myriads of beadlike ribosomes on its surface. They operate as miniature “forges,” where specific proteins are manufactured in accordance to “plans” that come in the form of messenger ribonucleic acid (mRNA) molecules that have been copied from the nuclear DNA. The origin of these many integrated cytoplasmic systems necessary for protein synthesis lacks an adequate evolutionary explanation. Much larger than ribosomes are mitochondria, which are visible in both the mycobiont and the phycobiont cytoplasm of Figures 2 and 9, and also in several other figures where they are labeled “11.” The mitochondria contain involved sequences of enzymes ingeniously arranged along a much-folded inner membrane (Figure 9, number “23”). Through a process called cellular respiration, this enzymatic array is involved in transforming the relatively low chemical bond energies of fuel molecules like glucose into the high-energy phosphate bonds of adenosine triphosphate (ATP). The high energy of the resulting ATP molecules is essential for empowering many endothermic chemical reactions involved in cellular repair, reproduction, and the synthesis of cytoplasmic polymers such as proteins. Some researchers argue that mitochondria are bacteria that once invaded other living cells. Smith and Brown (1985) provided evidence that mitochondria were instead created as organelles for each separate living kind. The dynamically complex series of coordinated mitochondrial macromolecules, each depending on the other for proper functioning of the cytoplasm, supports rapid creation. The cytoplasm is surrounded by a cytoplasmic membrane, which is called the plasmalemma, a labyrinthine barrier controlling the movement of chemical molecules into and out of the cell. The plasmalemma is visible in many of our figures as number “12” (e.g. Figure 2). It has the “mysterious function of allowing certain chemical substances free passage, while maintaining a closed-door policy to other materials” (Howe, 1966, p. 106). The intricate details of membrane structure and physiology, which command the attention of hundreds of researchers at numerous laboratories worldwide, fit with an intelligent design model.The Invaginations of the Mycobiont Plasmalemma Render Important Service
The invaginations of the fungal plasmalemmae are seen in Figure 9, number “22.” The plasmalemma of the phycobionts, on the other hand, is comparatively smooth. Smith (1973, p. 14) reported that the mycobiont plasmalemma convolutions generally occur where the fungus touches its algal partner (see also Peveling, 1973, p. 159). The invaginations provide an increased protoplast surface allowing high metabolic activity, and they indicate an “uptake of metabolic products” (Peveling, 1976, p. 23). Peveling thought they were an “adaptation,” promoting an exchange between symbionts (1973, p. 159). Her use of the term adaptation here would fit with the intelligent design view if one assumes that the Creator directly generated such “adaptations.” While avoiding the word adaptation, Brown and Wilson (1968) nevertheless made this same point: “The highly invaginated and tubular plasmalemma of the fungus points to a specialized molecular basis for exchange of material between the lichen components” (p. 239).A Storage Zone Lies Directly outside the Cytoplasm of Trebouxia
On the exterior of the plasmalemma, directly touching the cell wall, Trebouxia cells have a sizeable storage layer, which can be seen on Figure 2 as item “13.” Concerning this layer, Peveling (1976) wrote: “All substances observed between the plasmalemma and the cell wall may be considered as transferred metabolites” (p. 23). Photosynthetic products stored here are able to pass effectively from the photosynthetic algal partner to the fungus cell lying closely adjacent to it. Such ease of transfer is critical to the survival of lichens because the mycobionts depend on the phycobionts for their food supply. Storage Also Occurs in Droplets Formed inside the Trebouxia Plasmalemma Our photomicrographs (Figures 4, 5, and 7—see items labeled “21”) show these white droplets in the algal cytoplasm, as did those of Jacobs and Ahmadjian (1969, see pp. 228–229), who simply called them “storage droplets.” An anonymous referee of our paper suggested that perhaps these droplets are “evaginated vesicles transferring dissolved food to the storage layer,” a possibility that deserves further investigation. Thus two kinds of storage are associated with the cytoplasm: (1) a large depository of varying width between the plasmalemma and the algal cell wall, and (2) another system entailing numerous distinct white reservoirs at the edge of the cytoplasm, just inside the plasmalemma. These two cytoplasmic storage mechanisms, together with the reserves of lipid cached in the pyrenoid of the chloroplast and the starch reserves in the chloroplast stroma, encompass a large amount of reserve metabolic substrate. Workers who adopt the design view of origins would see this manifold storage program as an intelligent “overdesign,” enabling lichens to endure adverse environmental conditions.Nuclei and Nucleoli Are Present in the Phycobiont and the Mycobiont Cells
The nucleus in Trebouxia cells is seen clearly as “16” in Figures 5 and 7. A nucleolus is usually evident inside the algal nucleus. The chromosomes of the nucleus hold the DNA (genes), which control heritable characteristics. Nucleoli are repositories for mRNA molecules, which are produced from the DNA molecules and are then conveyed to the cytoplasm, where they govern protein manufacture. Nuclei of the mycobiont fungi are visible in Figures 2, 6, and 9 as number “16.” Concerning their size, Hale (1961, p. 7) wrote that “the nucleus [of the mycobiont] is exceedingly small, as is usual with the fungi.” The haploid chromosome number (base number of different kinds of chromosomes) in the nucleus of fungi varies between 2 and 8, depending on the species. Figure 12. Both mycobiont and phycobiont cells from the algal zone of Candelariella sp. Concentric bodies, often found in mycobiont cells, are visible (26) in the fungus cell, lower left. Present in both mycobionts and free-living fungi, concentric bodies evidently did not originate in mycobionts as a result of symbiosis, as evolutionists once thought. Various organelles from other figures are labeled and numbered here as previously: pyrenoglobuli (8), cell walls (14), and nucleus (16). Magnification is 6,000 X, while the scale bar is 15 micrometers.Concentric Bodies Occur in the Cytoplasm of Mycobiont Cells
Concentric bodies have also been historically called ellipsoidal bodies. They are found in the cytoplasm of lichen mycobiont cells, where they occur singly or in clusters, and may be surrounded by electron transparent halos. Groups of them lie in a matrix associated with the cell nucleus (Griffiths and Greenwood, 1972). They are small, having an “electron transparent core and two surrounding shells” (Peveling, 1973, p. 159), which have different electron densities (Jacobs and Ahmadjian, 1969, p. 231). Spherical in shape, the concentric bodies appear to possess radially arranged lamellae. With photomicrographs and sketches, Galun (1988a, see pp. 145–147) described the intricate structure of these rings and radial striations found in concentric bodies. They are evident at the lower left of our Figure 12, where we designate them as number “26.” Although the roles played by concentric bodies are not known with certainty, several suggestions have been made. Since they are often located in the proximity of the plasmalemma, they may be involved in membrane synthesis (Jacobs and Ahmadjian, 1969), possibly functioning as a kind of membrane repair kit. Like golgi bodies, they may also function in the movement of protein molecules. Ahmadjian (1993) suggested that they might even be viruses. In the 1970s, it was reported that concentric bodies had been discovered in 43 species of lichenized fungi and in two species of non-lichenized fungi, as well (Griffiths and Greenwood, 1972; Peveling, 1973). In 1993 Ahmadjian updated this tally, writing that concentric bodies had been identified in the mycobionts of about 100 species of lichens and in 11 different species of non-lichenized fungi. Galun (1988a) also confirmed the presence of concentric bodies in non-lichenized fungi by acknowledging that “the few free living [nonlichenized] fungi which contain concentric bodies are limited to ascomycetes” (p. 145). Classen et al. (2000) found concentric bodies present in a non-lichen ascomycete fungus parasitic on the pollen of the flowering plant Malva sylvestris.In the Lichen Literature, Is Evolution Usually Treated Like a Scientific Theory or Like an Origins Model?
The evolutionary idea that lichens and all life have descended gradually from a common ancestry over vast aeons is often espoused like some sort of religious/philosophical presupposition rather than being handled like a real scientific theory. Even after leading to false conclusions about scientific data, evolutionism is so very plastic that its devotees can quickly modify it to fit the conflicting new information. This demonstrates that evolution is an origins model, not a scientific theory. An illustration of this point exists in the history of lichenology. Evolutionism was part of a minor error that was corrected. We bring up one of these evolution-based errors, not to criticize the lichenologists involved, but to show the evolutionary basis of their error and to demonstrate that they reversed earlier ideas in order to preserve evolutionism. Two lichenologists once thought they had shown that concentric bodies are “unique” to mycobiont fungi (Jacobs and Ahmadjian, 1969, p.239). As a result of their early micrographic studies, they believed that concentric bodies are found only in the fungi of lichens (mycobionts) and are absent from non-lichenized fungi. Based on those data and on evolutionism, they suggested that concentric bodies were perhaps produced by evolution in the mycobionts while the condition of symbiosis was also being evolved, an idea that seemed plausible to them, based on their evolution origins model. In 1972, however, Griffiths and Greenwood reported discovering concentric bodies in two non-lichenized fungi. And, as stated earlier, it is now known that concentric bodies are widely present in non-lichenized fungi; they are not unique evolutionary innovations found only in mycobionts. This evolutionary error was rectified by Ahmadjian (1993, see p. 22). He did not hold evolutionary assumption accountable in any way, however, as he might have done, but simply originated a different evolutionary idea. Nor did he state that evolutionism had been shown to be somewhat inadequate in this situation. Instead, he completely reversed his original explanation, thereby creating a new evolution-based scenario. In this modified view, it was assumed that concentric bodies were probably widely present in the non-symbiotic ancestors of lichen fungi, who passed them on by evolution to their mycobiont descendants (Ahmadjian 1993). His “reversal” illustrates the manner in which evolutionism has little predictive power, frequently serving as just an afterthought that makes it applicable to almost any data. It also demonstrates that evolution is treated as a philosophical presupposition and is thereby considered to be unassailable, even when predictions based upon it turn out to be inaccurate. It has been said, and we think correctly so, that macroevolution is too plastic to qualify as a “scientific” theory. Scientific theories should be falsifiable, but the evolution theory is easily flexed to accommodate almost any outcome, making it safe from refutation. Renowned philosopher of science, Karl Popper, maintained that evolution is not a scientific theory but is instead a metaphysical proposition, because it cannot be subjected to falsification. His major philosophy, called “Falsificationism,” is the idea that “science advances by unjustified, exaggerated guesses followed by unstinting criticism” (Karl Popper web, 2006). Popper recognized that unfalsifiable theories, such as evolution, are somewhat “like the computer programs with no uninstall option that just clog up the computer’s precious storage space” (Karl Popper web, 2006). We agree with Popper’s assessment, and it is our personal opinion that the creation model likewise is “unfalsifiable.” Therefore, evolution and creation should both be classed as “origins models” and not as “scientific theories” (Armitage and Howe, 2004, pp. 250–251). This is not understood or practiced by many evolution-minded scientists and by many of the judges who have officiated in creation versus evolution court cases over the years. Unfortunately, such people have treated evolution as pure science while characterizing creation as totally “religious.”CONCLUSION
In forthcoming second part of this article, more cellular details of lichens will be presented. We will also continue to contrast evolution and creation in relationship to the origin of lichen ultrastructure. A close analysis of any living system supplies evidence in support of an origin by design.Acknowledgements
We are solely responsible for the comments and photographs in this essay, but we acknowledge with gratitude the generous assistance given to us in various ways. The following individuals gave us valuable editorial assistance: Stephen
B. Austin, Jerry Bergman, Wayne Frair, Lane Lester, E. Norbert Smith, and two anonymous reviewers. Peg Wesphalen secured many sources for us through interlibrary loan via top10paydasites.com. We are also grateful to Cindy Blandon for arranging the printed version of this paper. We express gratitude to the contributors to the CRS Research Fund and the Van Andel Creation Research Center Fund. Interest from these two endowments has helped finance various phases of our research.
Although disagreeing with the philosophical presuppositions of various lichenologists, we are nonetheless grateful for their detailed studies of God’s little lichens. With deep and final appreciation, we respectfully acknowledge the exceedingly skillful handiwork of the Creator, as reflected repeatedly in the ultrastructure of lichens.
References
CRSQ: Creation Research Society QuarterlyAhmadjian, V. 1993. The Lichen Symbiosis. John Wiley and Sons, New York, NY.
Ahmadjian, V. 2001. Trebouxia: Reflections on a perplexing and controversial lichen photobiont. In J. Seckbach (editor), Symbiosis, pp. 375–383. Kluwer Academic Publishers, the Netherlands.
Armitage, M.H., and G.F. Howe. 2004. Lichens at VACRC: Lichen surfaces under the electron microscope. CRSQ 4l:242–251.
Armitage, M.H., and G.F. Howe. 2006. Lichens in cross-section: Evidence for design and against macroevolution. CRSQ 42:252–264.
Borkhsenious, O.N., C.B. Mason, and J.V. Moroney. 1998. The intracellular localization of ribulose-1,5-biphosphate carboxylase/oxygenase in Chlamydomonas reinhardtii. Plant Physiology 116:1585–1591.
Brown, R.M. Jr., and R. Wilson. 1968. Electron microscopy of the lichen Physcia aipolia (Ehrh.). Journal of Phytology 4:230–240.
Classen, B., F. Amelunxen, and W. Blaschek. 2000. Concentric bodies in a parasitic fungus of Malva sylvestris (Malvaceae) pollen. Journal of Phytopathology 148:313.
Egan, Robert S. 2006. Lichen photobionts. http://avalon.unomaha.edu/lichens/ Bio%204350%20PDF/Photobionts.pdf (as of March 12, 2007).
Fisher, K.A., and N.J. Lang. 1971a. Ultrastructure of the pyrenoid of Trebouxia in Ramalina menziesii Tuck. Journal of Phycology 7:25–37.
Fisher, K.A., and N.J. Lang. 1971b. Comparative ultrastructure of cultured species of Trebouxia. Journal of Phycology 7:155–165.
Galun, M. 1988a. Lichenization. In M. Galun (editor), CRC Handbook of Lichenology, Volume II, pp, 153–172. CRC Press, Boca Raton, Fl.
Griffiths, H.B., and A.D. Greenwood. 1972. The concentric bodies of lichenized fungi. Archives of Microbiology 87:285–302.
Hale, M.E., Jr. 1961. Lichen Handbook: A Guide to the Lichens of Eastern North America. Smithsonian Institution Press, Washington, DC.
Howe, G.F. 1966. Cells, the amazing abode of life. In J.C. Monsma (editor), Behind the Dim Unknown, pp. 105–116. G. P. Putnam’s Sons, New York, NY.
Howe, G.F., and M. Armitage. 2002. Lichens: A partnership for life. CRSQ 39:81–88.
Howe, G.F., and M. Armitage. 2003. Lichens: A study in color. CRSQ 39:245–350.
Jacobs, J.B., and V. Ahmadjian. 1969. The ultrastructure of lichens. I. A general survey. Journal of Phycology 5:227–240.
Karl Popper web. 2006. Karl Popper’s 1934 bombshell. http://www.eeng.dcu. ie/~tkpw/ (as of March 12, 2007).
Lichtenthaler, H.K. 1968. Plastoglobuli and the fine structure of plastids. Endeavour 27:144–149.
Peveling, E. 1973. Fine Structure. In. V. Ahmadjian and M.E. Hale (editors), The Lichens, pp. 147–182. Academic Press, New York, NY.
Peveling, E. 1976. Investigations into the ultrastructure of lichens. In D.H. Brown, D.L. Hawksworth, and R.H. Bailey (editors), Lichenology: Progress and Problems, pp. 17–26. Academic Press. New York, NY.
Purvis, W. 2000. Lichens. Smithsonian Press, Washington, DC.
Smith, D.C. 1973. The Lichen Symbiosis. Oxford University Press, Oxford, UK.
Smith, E. C., and H. Griffiths. 1996. The occurrence of the chloroplast pyrenoid is correlated with the activity of a carbon dioxide-concentrating mechanism and carbon isotope discrimination in lichens and bryophytes. Planta 198:6–16.
Smith, T. L., and C. Brown. 1985. Perspectives on the origin of mitochondria. CRSQ 22:78–83.
Tschermak-Woess, E. 1988. The algal partner. M. Galun (editor), In CRC Handbook of Lichenology, Volume I, pp. 39–94.CRC Press, Boca Raton, Fl.
Tschermak-Woess, E. 1989. Developmental studies in trebouxioid algae and taxonomical consequences. Plant Systematics and Evolution 164:161–195.
Vaughn, K. C., E. O. Campbell, J. Hasegawa, H. A. Owen, and K. S. Renzaglia. 1990. The pyrenoid is the site of ribulose 1,5biphosphate carboxylase/oxygenase accumulation in the hornwort (Bryophyta: Anthocerotae) chloroplast. Protoplasma 156:117–129.